Biomechanics of the Spine in Sports and Prevention Considerations
Eeric Truumees, MD
Erik E. Swartz, PhD, ATC, FNATA
Dr. Swartz or an immediate family member serves as a board member, owner, officer, or committee member of the Athletic Training and Sports Health Care, the Journal of Athletic Training, and the New Hampshire Musculoskeletal Institute. Dr. Truumees or an immediate family member has received royalties from Stryker; has stock or stock options held in Doctor’s Research Group; has received research or institutional support from Globus Medical and Relievant; has received nonincome support (such as equipment or services), commercially derived honoraria, or other non-research–related funding (such as paid travel) from the North American Spine Society; and serves as a board member, owner, officer, or committee member of AAOS, AAOS Now, the Journal of Bone and Joint Surgery—American, the Journal of the American Academy of Orthopaedic Surgeons, the North American Spine Society, the Spine, and The Spine Journal.
Introduction
In sports, spine injuries occur across a wide spectrum from acute high-energy trauma to a chronic, repetitive overuse lesion. Appropriate diagnosis, management, and return to play for an athlete who has sustained a spine injury requires a reasonable understanding of the biomechanical mechanism of the injury. Whether the problem is low back pain that limits the athlete’s ability to train and compete or a devastating, destabilizing cervical spine dislocation with spinal cord injury (SCI), four aspects of the biomechanical milieu are important.
The first aspect to consider is what is the mechanism of injury? Each sport is associated with unique loading and injury patterns.1 Participation in any single sport may have acute or chronic effects on the spine, and in some cases, both. Examples of this include cycling, which can lead to high-energy impacts from crashes compared with the lumbar strain phenomena associated with the sustained posture common with endurance riding. Even acute injuries lie along a continuum of levels of imparted energy from repetitive micro-loading to devastating point loading. These issues may be interrelated because back and neck pain may impact subsequent training and readiness for competition. A second aspect to consider is which part of the spine is affected? Each region offers a very different biomechanical milieu. Understanding spinal biomechanics regionally and globally will assist the clinician in diagnosis, treatment, prevention, and rehabilitation strategies. Third, which tissue was predominantly affected? Although ligamentous sprain may be benign in some parts of the body, spinal ligament disruption may be devastating. Recognizing the variable impacts of sports-related injury on the muscles, joints, and bones of the spine offers the best first guide to treatment. Fourth, consider that each individual athlete’s personal characteristics affect his or her vulnerability to different injuries. A number of studies have shown differences in risk rates for male and female athletes. Similar differences exist for children, adolescents, adults, and older athletes. Understanding your player’s predisposing factors is another key step in proper diagnosis, rehabilitation, and the prevention of further injury.
The scope of this chapter and the available evidence prevent us from going into detail in every sport or every clinical circumstance. However, it is reasonable to use “best available evidence” and extrapolate from the data available to the unique combination of factors involved in the management of a given athlete’s injury.
Biomechanical Vulnerability by Tissue Type
The spine’s functions are both biomechanical and protective in nature. The biomechanical functions include transmitting body weight during ambulation and allowing for flexibility and motion of the extremities during activities of daily living. The spine also serves to protect the spinal cord; cauda equina; and along with the rib cage, major organs of the thoracic cavity. To understand the vulnerability of the spine and its contents to injury from sports, one must understand the material characteristics of the individual tissues involved and the ways in which the anatomic elements of the spine move together. Depending on the sport and the mechanism of injury, the spine may be subjected to compression, tension, shear, and rotational forces, often at the same time. Although the stability and weight-bearing ability of the thigh can be disrupted by a simple fracture of the femur, the spine requires several structures to be disrupted before stability is lost. This is not to suggest that incomplete disruption of tissues associated with a spinal level are not of clinical significance. In fact, the concept of a functional spinal unit (FSU) may be helpful in identifying spinal structures at risk.2 The FSU is a useful model for how these elements come together. The FSU consists of wo adjacent vertebral bodies, the interconnecting intervertebral disk, the articulating facet joints, and the segmental components of ligaments and muscles.
Injury will occur when those extrinsic forces exceed the intrinsic strength of the spine and its encasing soft tissues. Because these tissues work together as a unit, the strength and function of the muscles impact the likelihood of disk or bone injury. For example, coactivation of adjacent trunk muscle units may help direct forces along an efficient pathway. In this way, forces are not focused on a vulnerable area, and tissue failure may be avoided.
Soft Tissue Injuries
As with sports injuries to the bone and joints of the spine, most musculoligamentous injuries occur through indirect loading. Direct blows rarely lead to bony injuries in the spine but occasionally affect the soft tissues.3 Indirect loading injuries can occur either with acute, high-energy mechanisms or with chronic overuse, high-repetition mechanisms. Typically, acute musculoligamentous injuries to the spine are caused by violent rotational or bending forces.4 Chronic musculoligamentous injury is more common and often results from sustained high-repetition activities. Although nondestabilizing, these injuries can result in debilitating symptoms.4 Rowing, for instance, has a 22% incidence of back injury and a 9% incidence of rib cage injury from continuous, repetitive motion, particularly through the pull of the serratus anterior.5
Although musculoligamentous injuries certainly can and do occur in the cervical and thoracic spine, lumbar strain is the most common cause of low back pain in athletes. Here, too, a spectrum of injury from simple muscle bundle irritation without disruption to intrasubstance tearing of the muscle fibers or fascia is possible. Injury to the various spinal ligaments, or sprains, may also occur. In the cervical spine, ligament disruption to the transverse atlantal ligament, for example, resulting from focal hyperflexion of the upper cervical spine, can lead to a devastating neurologic injury.6 More common, and more benign, are spinal ligamentous injuries involving the supra- and interspinous ligaments of the thoracolumbar region. These ligaments typically function as elastic stabilizers for the spinal segment and assist in extension. They are typically injured with abrupt segmental flexion.
When a significant rotational moment is added to a flexion injury, additional damage to the lumbar facet joint capsules or thoracic costovertebral joints may be seen. Higher energy trauma may lead to additional injury to the spinal ligaments, such as the anterior and posterior longitudinal ligaments (ALL and PLL), but these are almost always in combination with disk or bone injuries and discussed elsewhere.
Joint Injuries
Critical components in the biomechanics of the FSU come from the articulations, which include the intervertebral disk and the facet joints. As with musculotendinous injury, disk injuries in sports span a wide spectrum from traumatic, massive annulus disruption to repetitive traumas leading to degeneration. The zygapophyseal joints (facets) may be injured at any level of the spine. The relative risk, causative mechanisms, and implications on spinal stability vary by region and are discussed later.7
Mechanically, the intervertebral disk has two main functions: axial load transmission (a burden carried mainly by the nucleus pulposus) and ROM, particularly rotation (carried out by the annulus fibrosis). Hence, compromise of one structure, the nucleus or annulus, through a distinct biomechanical pattern can lead to injurious effects in the other structure. For example, most disk herniations are thought to occur after a series of repetitive traumas has created small rents and tears between annular fibers. Later, asymmetric axial loads on the disk may force a portion of the nucleus pulposus to migrate through the zone of
annular weakness and into the spinal canal. Because the posterior longitudinal ligament is rarely injured in this context, the herniation is more typically posterolateral.8 Symptomatic disk herniation are more common in the lower lumbar spine than in the upper lumbar and thoracic spine.9 When they do occur in the thoracic spine, they are typically below T8.9,10 Risk for these injuries increases for patients in their fourth and fifth decades. With higher energy trauma in younger patients, the PLL and the annulus are acutely and massively disrupted, allowing a larger portion of disk material to enter the canal centrally. In professional sports, upper lumbar and thoracic disk injuries are not particularly common and are typically attributed to contact injuries such as blocking and tackling.11
annular weakness and into the spinal canal. Because the posterior longitudinal ligament is rarely injured in this context, the herniation is more typically posterolateral.8 Symptomatic disk herniation are more common in the lower lumbar spine than in the upper lumbar and thoracic spine.9 When they do occur in the thoracic spine, they are typically below T8.9,10 Risk for these injuries increases for patients in their fourth and fifth decades. With higher energy trauma in younger patients, the PLL and the annulus are acutely and massively disrupted, allowing a larger portion of disk material to enter the canal centrally. In professional sports, upper lumbar and thoracic disk injuries are not particularly common and are typically attributed to contact injuries such as blocking and tackling.11
In the cervical spine, the articular pillars, which include the lateral masses and facet joints, carry a far greater percentage of the axial load passing through the segment than in the thoracolumbar spine.12 The inherent stability conferred by the rib cage offers relative protection to the thoracic facets, but injuries still may be seen. With slight medial angulation, the thoracic facets are oriented vertically in the coronal plane. This orientation limits the thoracic spine’s flexion and extension but allows for lateral bending and rotation. Excessive or rapid flexion or extension may lead to facet joint fracture. Lumbar facet joint injury is more common.13 These lesions are thought to occur when a rotational injury overwhelms the stabilizing effect of facet capsule.
Bony Injuries
Sport activities can cause a variety of fractures in the cervical, thoracic, and lumbar spine. Some of these injuries are more aptly included with musculotendinous injuries such as isolated transverse or spinous process fractures. Sadly, however, destabilizing fractures and fracture-dislocations are also seen after athletic pursuits. As with the soft tissue and joint injuries already discussed, most of these injuries occur through indirect loading. Direct injury to a spinous process or the coccyx leading to fracture can also occur, but these are typically more benign injuries in terms of their mechanical impact on spinal stability.17,18 Sports-related bony injury from indirect loading is divided into acute fractures and repetitive microtrauma or stress fractures.
In the thoracolumbar spine, stress fractures occurring in spinous processes, transverse processes, pars interarticularis, or ribs can result from overuse activities. Although a number of factors interact to cause these injuries, three are most important biomechanically: degree of loading, frequency of loading, and bone quality.19 Understandably, as the degree (rate or force) or frequency increases, even in the presence of healthy bone, risk of stress fracture increases. Prevention strategies therefore require that one or more of these factors be addressed.
Spinous process avulsion fracture can occur either through acute injury or through repetitive muscular shear injury. These injuries are most common in the lower cervical or upper thoracic spine, where they are termed clay-shoveler fractures. This region may be affected more frequently in sports emphasizing the overlying shoulder girdle musculature. Single and multiple spinous process avulsions have been reported in a number of sports, including golf, rock climbing, baseball, and wrestling.20,21,22
More common are defects in the pars interarticularis. Such spondylolysis is most common at L5 and is a common cause of low back pain in younger athletes. In one study, low back pain in young athletes was thought to be related to a stress fracture 48% of the time.23 Spondylolysis can occur more cranially in the spine. These injuries are rare in thoracic spine because the limited arc of flexion and extension is less likely to stress the pars interarticularis. A finite element investigation concluded that multilevel spondylolysis was more likely due to genetic than mechanical factors.24 A biomechanical analysis has suggested that athletes with cervical spondylolysis not return to contact sports.25
Stress fractures of the sacrum are uncommon, mostly typically seen in runners and volleyball players.26 Repetitive impact loading is thought to be the most significant risk factor. The role of decreased bone density in the incidence of these injuries continues to be debated.27
Acute fractures are more severe injuries, particularly in the cervical spine, and are typically caused by higher energy spinal loading activities, such as skiing, rugby, and football. These often involve a common mechanism, an axial load, and are discussed in the next section.
In the thoracic spine, axial loading and flexion forces may cause compression fractures, in which the anterior column fails while the posterior bone and ligamentous structures remain intact. Because of the inherent stability from the ribs and sternum, thoracic compression fractures rarely require operative treatment.7,28 In an athletic population, adequate bone healing and muscle
rehabilitation have typically occurred to allow return to sport by 3 months.29
rehabilitation have typically occurred to allow return to sport by 3 months.29
With higher levels of energy imparted and the addition of flexion and rotational moments, more severe fracture patterns such as burst, translation rotation, and flexion-distraction fracture can be seen.30,31,32 These injuries are more likely to lead to true spinal destabilization and confer a higher risk of SCI.
Spinal Cord Injury
The most feared aspect of any athletic injury to the spine is the possibility of damage to the neurologic tissues (the cauda equina, the nerve roots, and the spinal cord, in particular). The overall incidence of SCI varies drastically by anatomic region and sport type. However, sports are reported to be the second most common cause of SCI in patients younger than 30 years of age.33 The most common mechanism of injury in sport leading to a cervical SCI is an axial load, such as with head-initiated strikes in diving, football, skiing, and horseback riding.34,35
Regional Biomechanics of the Spine
Just as each tissue type carries a different risk of injury and different types of injury, the regional differences in pathomechanics of the spine offer different opportunities for prevention.
Cervical
In terms of potentially devastating, sports-related spine trauma, head and neck injuries typically get the most attention. Because the mechanisms for acute and chronic head or neck injury often share the common mechanism of head-initiated contact, a variety of prevention efforts are underway. Many of these efforts seek to address the pathomechanics of the most common injury patterns. In the 1970s, better helmets led to an increased use of head during tackling. This “spear tackling” led to an epidemic in which as many as 70% of nonfatal cervical spine injuries in football resulted from head impacts.36,37
From a biomechanical perspective, the relatively small size of the cervical spine relative to the size of the head means that only 20% of its overall stability comes from the osteoligamentous structures.38 The other 80% arises from the strong anterior and posterior neck muscles. As such, there is significant overlap between cervical ROM and neck strength on the one hand and the types and severity of head and neck injuries on the other. Theoretically, for example, athletes may reduce their head acceleration in an impact by contracting their cervical musculature, which serves to increase the effective mass of their head.39,40 Unlike a whiplash scenario in motor vehicle accidents, athletic collisions in which the neck stops the head rarely impart enough energy to cause injury relative to those in which the neck must stop the torso.41 As mentioned, most cervical spine injuries occur in conjunction with a head strike. So, appropriate prevention and return to play recommendation require an understanding of the dynamics of cervical spine injury.42
Initially, the common hypothesis was that head movement caused the spine to exceed its ROM. This, in turn, lead to a cervical spine injury.43 However, in 1972, Roaf first suggested a mechanistic model based on forces and bending moments acting on the cervical spine.44 Later, seminal studies by Bauze and Ardran and Nightingale et al demonstrated that severe cervical spine injuries, including bilateral facet dislocations and compression-flexion injuries, could occur within the normal arc of motion.45,46
More recently, these models have been updated with the concept of buckling, which arose to explain how multiple, noncontiguous and disparate injuries can occur.42,46,47 In experimental studies, cervical spine buckling has been shown to precede injury. This buckling forces the spine into a complex shape with some regions of pure compression, compression-flexion, and compression-extension.42,46,48,49,50 This model explains why high-energy collisions are not needed to create devastating injury patterns (Figure 2-1). To stop the moving torso, depending on the position of the head and the alignment of the cervical spine, only a small percentage of body weight is needed to disrupt the cervical spine. In one study, only 16 kg of mass at a velocity of 3.1 m/s was required.46,51
There are three important time points after these head-first impacts: the initial head-strike and the force vectors on the cervical spine that follow moments later followed by the continuing movement of the body. In an unhelmeted impact to the top of the skull, a large, 8-kN force is generated but dissipates within milliseconds. Although there is initially no force on the cervical spine, a few milliseconds later, the still moving torso will exert profound compression between the head and body across the cervical spine. Helmets markedly reduce head impact forces to under 4 kN but do not protect the neck because cervical spine injury occurs shortly after head strike. In fact, certain helmets may increase the chance of cervical spine injury by holding the head to the impact surface longer.42 Referred to as “pocketing,” upon impact, the head sinks into the helmet’s interior padded surface rather than being allowed to deflect off and away from the impact surface.51 Then, as
the torso keeps moving, the “held” head forces the cervical spine into positions of hyperflexion or extension, depending on the point of impact of the skull. With or without a helmet, the position of the neck at the moment of impact has a critical effect on the chance, severity, and type of cervical spine injuries seen. Typically, a position of slight flexion is thought to be more dangerous than facial or back of the head impacts.46,51,52
the torso keeps moving, the “held” head forces the cervical spine into positions of hyperflexion or extension, depending on the point of impact of the skull. With or without a helmet, the position of the neck at the moment of impact has a critical effect on the chance, severity, and type of cervical spine injuries seen. Typically, a position of slight flexion is thought to be more dangerous than facial or back of the head impacts.46,51,52
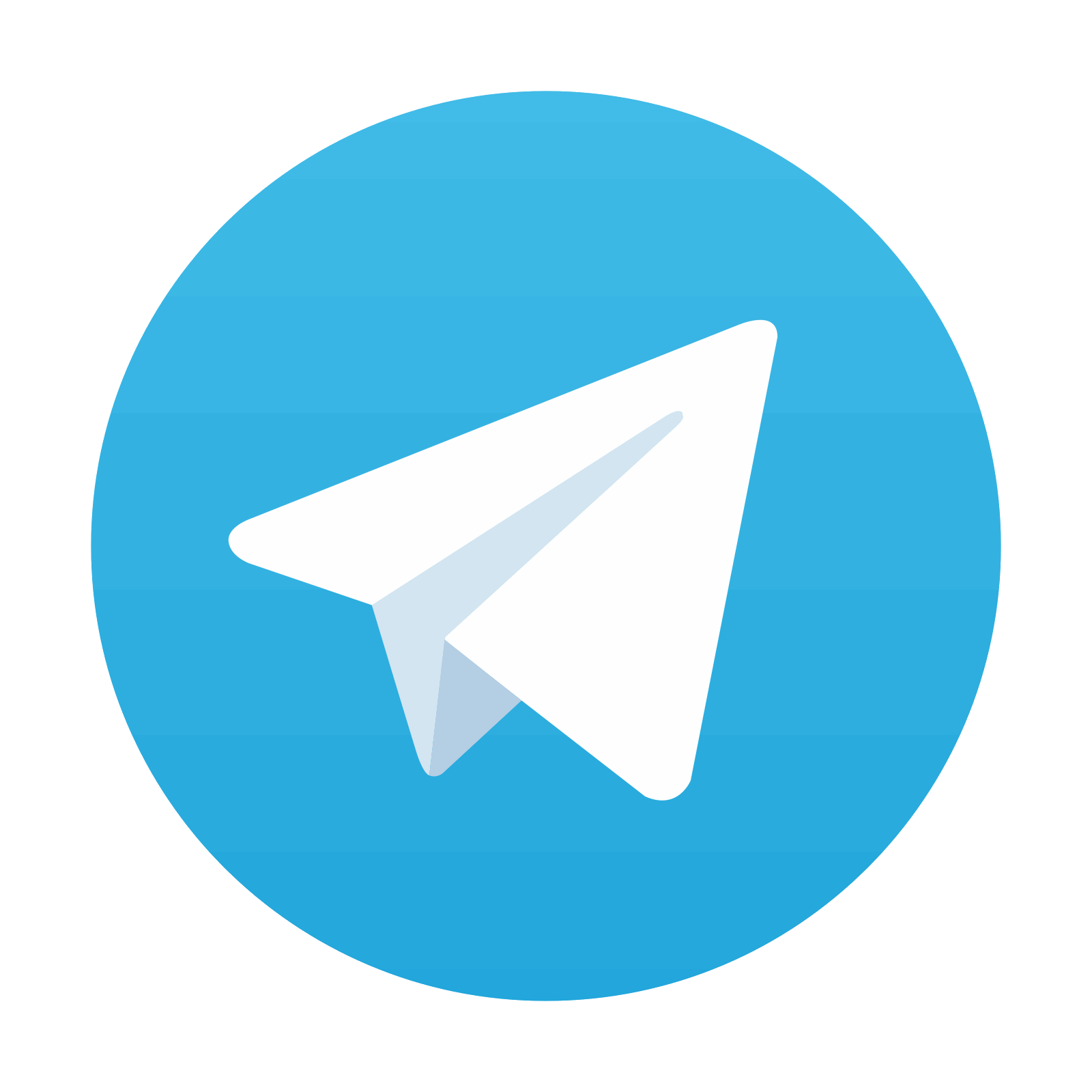
Stay updated, free articles. Join our Telegram channel

Full access? Get Clinical Tree
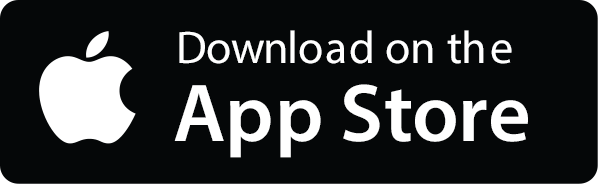
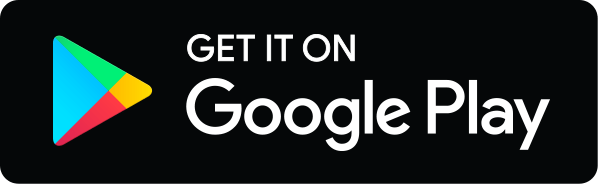