Biomechanics of the Foot and Ankle
Richard J. de Asla, MD
Dr. de Asla or an immediate family member serves as a paid consultant to or is an employee of Arthrex, Inc. and has stock or stock options held in Pfizer.
ABSTRACT
Understanding biomechanics and functional anatomy of the foot and ankle is mandatory if there is to be any meaningful attempt at formulating new ways of addressing its pathoanatomy. This chapter provides an introduction to foot and ankle biomechanics and functional anatomy as a basis for treating its disorders.
Introduction
The foot is a marvelous mechanical structure. Its unique anatomy and biomechanics allow it to play several seemingly conflicting roles. During push-off, it is a rigid lever arm for efficient propulsion. In stance, it is a stable platform for balance. It is also a part-time shock absorber that adeptly navigates uneven surfaces and terrain. The average person’s foot is remarkably durable, logging more than 100 million steps during an average lifetime.
Since the development of advanced imaging, knowledge of foot and ankle biomechanics has increased tremendously.1,2,3,4,5 This improved understanding of how the foot works provides better insight into disorders of the foot and ankle, which are often biomechanically based.
A thorough understanding of the biomechanics and functional anatomy of the foot and ankle is mandatory to ensure successful treatment of patients and the development and advancement of new procedures.
Structural Anatomy
The foot is divided into three regions: the forefoot, midfoot, and hindfoot. The tarsometatarsal joints (or Lisfranc joint complex) separate the forefoot from the midfoot, and the talonavicular and calcaneocuboid joints (transverse tarsal or Chopart joint) separate the midfoot from the hindfoot.
In the forefoot, the metatarsals are unique in that they are the only long bones in the body to support weight perpendicular to their long axis. In most feet, the first metatarsal is somewhat shorter than the second, with a progressive cascade of shortening from the second to the fifth metatarsal. This metatarsal break angle encourages the foot to supinate during push-off. In the sagittal plane, all metatarsals incline to some extent; the first metatarsal has the highest inclination angle (range 15° to 25°), and the remaining metatarsals demonstrate decreasing inclination angles from medial to lateral. Alterations and subtleties in the length and position of the metatarsals affect loading patterns that may influence alignment and contribute to the development of painful callosities, metatarsalgia, and metatarsophalangeal joint pathology.
The first, fourth, and fifth metatarsals have mobility in the sagittal plane, whereas the second and third metatarsals have relatively fixed positions. The lesser metatarsal bases are connected by a series of plantar metatarsal ligaments. No such connection exists between the base of the first and second metatarsals. The absence of an intermetatarsal ligament provides the first metatarsal a degree of mobility in the transverse plane not afforded to the lesser metatarsals. This anatomic feature may play a role in the development of hallux valgus deformities.
The forefoot is connected to the midfoot through the Lisfranc joint complex. Here, the cross-sectional wedge-shaped cuneiforms and metatarsal bases form a transverse arch across the midfoot, enhancing stability in the coronal plane (Figure 1). Additionally, the base of the second metatarsal is recessed proximally between the medial and lateral cuneiforms, providing stability in the horizontal plane. Stability is further enhanced by a series of very strong plantar tarsometatarsal ligaments and a short, thick interosseous ligament that courses between the lateral aspect of the medial cuneiform and medial aspect of the second metatarsal base. This interosseous ligament is often referred to as the Lisfranc ligament. Disruption of the midfoot complex may result in an avulsion fracture off the base of the second metatarsal. The bony fragment, still attached to Lisfranc ligament, can be seen between the bases of the first and second metatarsals on plain radiographs—the so-called fleck sign.
The midfoot contains the navicular, the cuboid, and three cuneiform bones. These five bones are relatively immobile with respect to one another and provide a mechanical link between the hindfoot and the more
mobile forefoot. The midfoot allows for the safe passage of neurovascular structures and tendons as they course from the leg to the foot. The disk-shaped navicular bone has a convex anterior surface and a concave posterior surface—both of which are covered with cartilage. Small vessels enter the navicular dorsally from the dorsalis pedis artery and medially from the posterior tibial artery. Blood supply to its central portion is relatively sparse, and certain foot types may accentuate shear across this portion of the bone.6 The navicular is also the primary attachment site of the tibialis posterior tendon. These features may combine to make the navicular bone relatively susceptible to stress fracture and poor healing.
mobile forefoot. The midfoot allows for the safe passage of neurovascular structures and tendons as they course from the leg to the foot. The disk-shaped navicular bone has a convex anterior surface and a concave posterior surface—both of which are covered with cartilage. Small vessels enter the navicular dorsally from the dorsalis pedis artery and medially from the posterior tibial artery. Blood supply to its central portion is relatively sparse, and certain foot types may accentuate shear across this portion of the bone.6 The navicular is also the primary attachment site of the tibialis posterior tendon. These features may combine to make the navicular bone relatively susceptible to stress fracture and poor healing.
The midfoot is separated from the hindfoot by the talonavicular and calcaneal cuboid joints, known collectively as the transverse tarsal joint or Chopart joint. The talonavicular joint is a ball-and-socket-type joint. The socket that receives the talar head is deepened by the anterior and middle facets of the calcaneus, the calcaneonavicular slip of the bifurcate ligament, and the superomedial and inferior calcaneal navicular ligaments (spring ligament). The superomedial component of the spring ligament serves to suspend the head of the talus, functioning like an anatomic hammock. This “acetabulum pedis” allows for transverse, sagittal, and longitudinal planes of motion and plays a vital role in foot biomechanics (Figure 2). Any motion of the talonavicular joint or subtalar joint also involves motion at the calcaneocuboid joint. Maximum congruency of the calcaneocuboid joint is achieved when the hindfoot is in varus and the forefoot is supinated. This is the position the foot assumes with push-off.
The hindfoot consists of the calcaneus and the talus. Their articulation forms the subtalar joint as the talus sits “side saddle” over the superomedial aspect of the calcaneus. The subtalar joint consists of three separate articulations, or facets. More than 90% of all tarsal coalitions occur at either the anterior facet (calcaneonavicular coalition) or the middle facet (talocalcaneal coalition). For simplicity’s sake, subtalar motion is often depicted as inversion and eversion around a mitered hinge. In reality, subtalar motion is quite complex, is difficult to measure, and includes rotational motions and translations in multiple planes. The subtalar joint is stabilized by the deltoid ligament, the interosseous and cervical ligaments, and a series of lateral ligaments and structures. These lateral stabilizers include the calcaneofibular ligament (CFL), the lateral talocalcaneal ligament, and the inferior extensor retinaculum. Because of its role as a subtalar joint stabilizer, the inferior extensor retinaculum is often used in Broström-type lateral ankle ligament reconstruction procedures7,8,9 (Figure 3).
The body of the talus resides in a bony housing created by the articulation between the distal fibula and tibia. The mortise is formed by the tibial plafond and medial and lateral malleoli. Composed of the talus,
distal tibia, and fibula, the ankle joint includes three articulations: tibiofibular, tibiotalar, and talofibular. The tibiofibular joint represents the inferior extent of the lower extremity syndesmosis. The syndesmosis is stabilized by four ligaments: the anterior inferior tibiofibular ligament, the posterior inferior tibiofibular ligament, the transverse tibiofibular ligament, and the interosseous tibiofibular ligament. Occasionally, a thickened accessory slip of the anterior inferior tibiofibular ligament (Bassett ligament) may insert too distally on the fibula, causing symptoms as it impinges against the anterolateral aspect of the talar dome. The transverse tibiofibular ligament originates on the posterior aspect of the fibula and extends to the posterior margin of the medial malleolus. In between, it forms the posterior labrum, which effectively deepens the tibiotalar joint. The syndesmosis allows fibula rotation and proximal migration when the wider anterior aspect of the talar body rotates into the ankle mortise during dorsiflexion. This relationship also allows the fibula to share approximately 16% of the axial load transmitted across the ankle.10,11,12
distal tibia, and fibula, the ankle joint includes three articulations: tibiofibular, tibiotalar, and talofibular. The tibiofibular joint represents the inferior extent of the lower extremity syndesmosis. The syndesmosis is stabilized by four ligaments: the anterior inferior tibiofibular ligament, the posterior inferior tibiofibular ligament, the transverse tibiofibular ligament, and the interosseous tibiofibular ligament. Occasionally, a thickened accessory slip of the anterior inferior tibiofibular ligament (Bassett ligament) may insert too distally on the fibula, causing symptoms as it impinges against the anterolateral aspect of the talar dome. The transverse tibiofibular ligament originates on the posterior aspect of the fibula and extends to the posterior margin of the medial malleolus. In between, it forms the posterior labrum, which effectively deepens the tibiotalar joint. The syndesmosis allows fibula rotation and proximal migration when the wider anterior aspect of the talar body rotates into the ankle mortise during dorsiflexion. This relationship also allows the fibula to share approximately 16% of the axial load transmitted across the ankle.10,11,12
![]() FIGURE 3 The illustrations depict a modified Broström procedure. Note that a portion of the inferior extensor retinaculum is mobilized for incorporation into the repair. |
The ankle is stabilized by the inherent bony configuration of the mortise,13 as well as the medial and lateral ligament complexes. One author compared the articular surface of the talus with a truncated cone in which the medial aspect is oriented toward the apex and the lateral aspect is oriented toward the base. Therefore, this cone has a smaller medial radius and a larger lateral radius14 (Figure 4). The articular surface of the talus is also narrower posteriorly than anteriorly. When the ankle is dorsiflexed, the widened anterior portion of the talus fills the mortise more effectively, improving bony stability. In plantar flexion, the bony contribution to stability decreases and the surrounding ligaments assume
an increased role. The deltoid ligament complex is well configured to stabilize the medial aspect of the ankle where the apex of the deltoid meets the apex of the cone. The deltoid ligament complex is divided into two anatomically distinct layers: superficial and deep. The superficial layer is fan-shaped and has no discrete bands, although it has been divided into as many as five components. The anatomically separate deep layer is short and thick and divided into two distinct ligaments: the anterior and posterior tibiotalar ligaments. Both layers act to resist valgus talar tilting and act as secondary restraints to anterior translation of the talus. The deep layer is the primary restraint to talar external rotation.
an increased role. The deltoid ligament complex is well configured to stabilize the medial aspect of the ankle where the apex of the deltoid meets the apex of the cone. The deltoid ligament complex is divided into two anatomically distinct layers: superficial and deep. The superficial layer is fan-shaped and has no discrete bands, although it has been divided into as many as five components. The anatomically separate deep layer is short and thick and divided into two distinct ligaments: the anterior and posterior tibiotalar ligaments. Both layers act to resist valgus talar tilting and act as secondary restraints to anterior translation of the talus. The deep layer is the primary restraint to talar external rotation.
The lateral ankle ligament complex is configured more broadly to accommodate a wider radius and larger arc of rotation. The lateral ankle ligament complex comprises the anterior talofibular ligament (ATFL), the CFL, and the posterior talofibular ligament. Lateral ankle stability depends on the orientation of the fibers of each of these ligaments, which changes with ankle position. The ATFL is a thickening of the anterolateral ankle joint capsule that is visible from the articular side of the capsule. Among all lateral ligaments, the ATFL has the lowest load to failure but the highest strain; it lengthens the most before failure. When the foot is plantarflexed, the fibers of the ATFL orient parallel to the leg, providing collateral restraint to inversion. The CFL is a distinct extracapsular, cordlike ligament that spans the ankle and the subtalar joints. This ligament is always perpendicular to and stabilizes the subtalar joint.
Clinical Biomechanics
At its most basic level, the foot can be conceptualized as a tripod. In stance, weight is distributed collectively between the head of the first metatarsal, the heel, and the four lesser metatarsal heads. Malalignment and alterations in biomechanics may disrupt this balance, resulting in painful conditions (Figure 5). For example, a cavus foot type is more prone to increased loading under the first metatarsal head and lateral aspect of the foot. A patient with a dorsiflexion malunion of a metatarsal fracture is at increased risk for a painful callus under another metatarsal head. A hallux valgus deformity may lead to second metatarsal phalangeal joint overload, plantar plate attenuation, and eventual crossover second toe deformity.17 Furthermore, foot deformity and position may ultimately affect more proximal joints, as seen in patients with planovalgus foot deformities. The rotational forces created by the planovalgus foot may eventually result in attenuation of the deltoid ligament and valgus tilting of the talus. When a foot and ankle surgeon attempts to correct deformity, the tripod concept must be kept in mind.
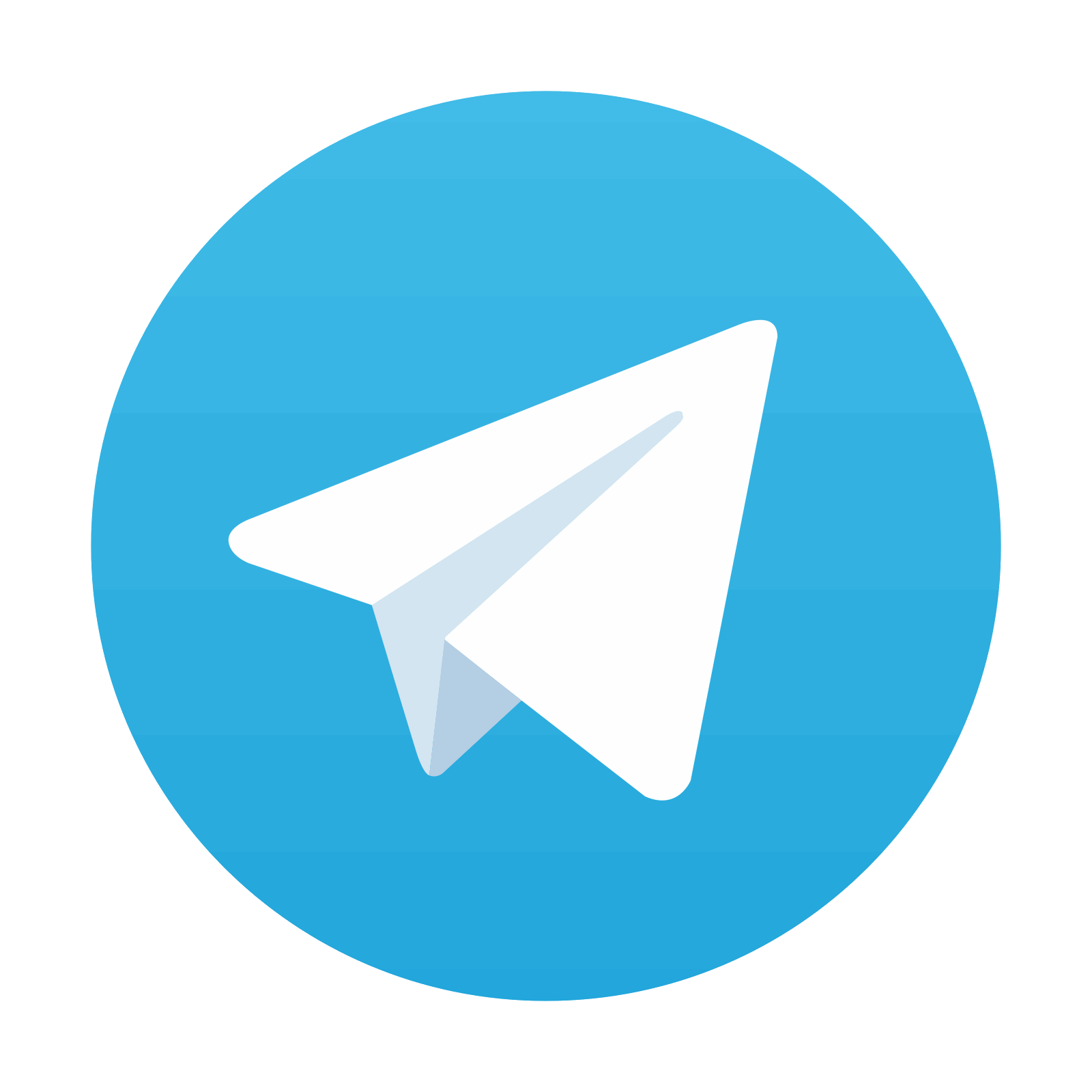
Stay updated, free articles. Join our Telegram channel

Full access? Get Clinical Tree
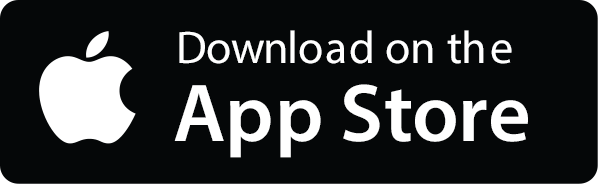
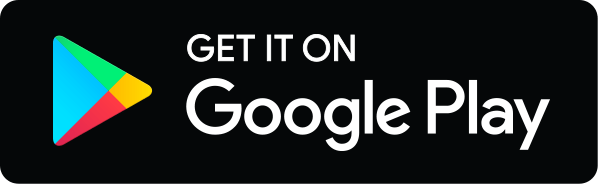