Biomechanics of External Fixation
James E. Orsak
J. Tracy Watson
Introduction
Perhaps one of the biggest advantages of using external fixation is the ability to modulate the healing environment throughout the course of treatment. From Julius Wolff’s work in the late 1800s, we know that bone adapts to its mechanical environment. An external fixator is a structural substitute for intact bone and is thus greatly influenced by biomechanical principles. The piezoelectric properties of bone as demonstrated by Fukada and Yasuda in the 1950s ensure that the bone architecture is enhanced by mechanical loading. Julius Wolff’s work was refined by Dr. Harold Frost in the 1960s who brought attention to an often overlooked point that bone adapts to dynamic loads as opposed to static loads which implies that the role of ambulation or weight bearing may be key to the healing process. This point was further emphasized by Giovanni DeBastiani in the 1980s. DeBastiani was an Italian physiologist whose study of bone healing led to the coining of the term dynamization which is the controlled destabilization of the external fixator after an initial period of absolute rigidity to allow the bone to begin sharing the axial load.
Principles of Dynamization
There are two phases to the dynamization process: The first phase is a controlled micromotion of less than 2 mm initiated 2 weeks after surgery, and the second phase is a complete axial collapse initiated at approximately 6 weeks (Figure 3.1). DeBastiani’s external fixator was designed to restrict bending movement while allowing axial sliding through a telescopic tube (Figure 3.2A and B). His design allowed for progressive load sharing between the external fixator and the bone to make full use of Wolff’s law. A comparison of a rigidly fixed versus dynamized fracture results is shown in Figure 3.3.
![]() Figure 3.2. Examples of DeBastiani’s dynamic axial external fixator with silicone cushion collar for micromotion (A, B). |
The precise amount of dynamization during the controlled micromotion phase was studied by Drs. Goodship and Kenwright from Oxford University. Their animal study using sheep found that a fracture gap motion of 0.5 mm is optimal for stimulation of callus growth whereas motion greater than 2 mm was detrimental. In a follow-up study on humans, they were able to demonstrate an improvement in the healing time of 4 to 5 weeks with the dynamized patients.
The Effects of Cyclic Microshear on Fracture Healing
From the principles of dynamization it has long been believed that while axial loading is beneficial to fracture healing, shearing forces have classically been considered to be detrimental to fracture healing. In their clinical studies, Sarmiento et al. observed that fracture treatment with functional casts and braces that allow shearing motion between the fragments produces more abundant callus and unites more rapidly and consistently than fractures that are inherently stable. More recently, Blenman et al., using two-dimensional finite element analysis of a healing osteotomy, proposed that shear stresses at the healing fracture site encouraged cellular proliferation and promoted ossification.
However, there is little experimental data to support these clinical and mathematical findings. Yamagishi and Yoshimura concluded that shearing force was important in the production of a pseudarthrosis. In these experiments, the shear forces were poorly controlled as the force was applied with springs. Additionally, the shear forces were combined with a distraction force. In a study to determine the effects of controlled intermittent cyclic microshear, Sauer et al. measured callus formation and density in osteotomized rabbit tibiae that experienced controlled shear versus a control group. The results showed that the osteotomies experiencing the microshear forces produced more abundant and denser callus formation corresponding to increased torsional strength and stiffness (Figures 3.4 and 3.5). Their findings, contrary to popular belief, showed that within a specific range microshear forces can actually be beneficial to fracture healing.
Modes of External Fixation
In contrast to dynamic loading that produces callus formation (also known as secondary healing), there are the principles of
rigid fixation (resulting in primary healing) popularized by the Arbeitsgemeinschaft für Osteosynthesefragen (AO) Foundation as well as Sir John Charnley who published his work on compression arthrodesis in 1953. Since external fixation is positioned away from the bone, there is a natural element of flexibility due to the bending stiffness of the materials used and therefore the inherent design of the external fixator lends itself well to dynamic loading. It is by adding compression elements to the design that the biomechanics of the external fixator can be modified from a secondary healing approach to a primary healing device. Charnley’s external fixator utilized threaded rods and wing nuts to provide compression to transfixion pins to the point of absolute stability at the fracture site (Figure 3.6).
rigid fixation (resulting in primary healing) popularized by the Arbeitsgemeinschaft für Osteosynthesefragen (AO) Foundation as well as Sir John Charnley who published his work on compression arthrodesis in 1953. Since external fixation is positioned away from the bone, there is a natural element of flexibility due to the bending stiffness of the materials used and therefore the inherent design of the external fixator lends itself well to dynamic loading. It is by adding compression elements to the design that the biomechanics of the external fixator can be modified from a secondary healing approach to a primary healing device. Charnley’s external fixator utilized threaded rods and wing nuts to provide compression to transfixion pins to the point of absolute stability at the fracture site (Figure 3.6).
Through the mechanical advantage of threaded components, external fixators convert the rotational forces of threaded nuts to linear forces that move bone segments in compression or distraction. There are three basic biomechanical modes to external fixation: Compression, distraction, and neutralization. The choice of which mode to use may depend on the treatment objective, fracture pattern, fracture location, or soft tissue condition. The compression mode is often used for joint arthrodesis or simple fractures to impart stability to bone ends. If the fracture pattern is highly comminuted, then a neutralization mode is used to prevent limb shortening at the fracture site. The distraction mode is used for osteogenesis or callotasis method for limb lengthening as well as for ligamentotaxis to provide stability to intra-articular fracture fragments.
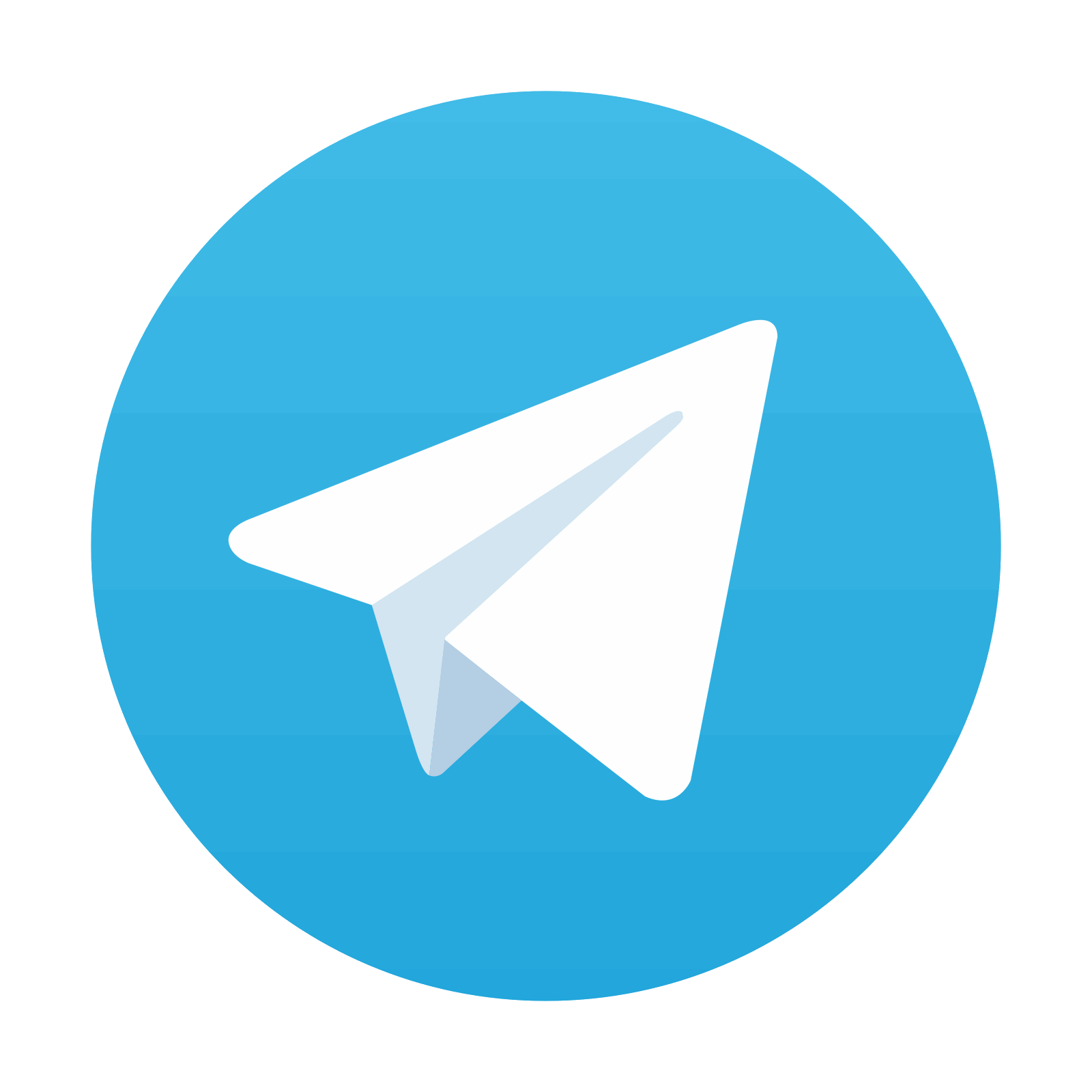
Stay updated, free articles. Join our Telegram channel

Full access? Get Clinical Tree
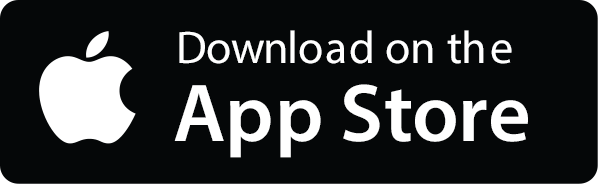
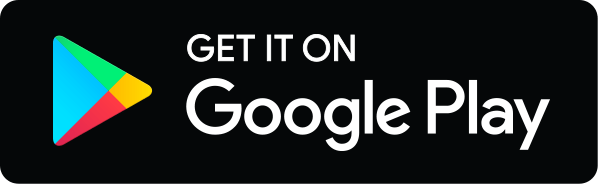