Biomechanics of Aging Spine
Hassan Serhan
Vijay Goel
From a biomechanical perspective, several skeletal components working in harmony allow us the day-to-day human experiences of walking, running, and, in some exceptional cases, hitting a perfect golf swing.1 Our spine happens to be one of the critical components. The individual vertebrae, interconnecting ligaments, shock-absorbing disks, and facet joints all play a role in the performance of these activities. However, as we age, each of these components starts to deteriorate, evidenced by its poor biomechanical characteristics. Ultimately, degenerative cascades result in diseases like osteoporosis, spinal stenosis, spondylolysis, and spondylolisthesis. The objective of this review is to provide: (a) a very brief introduction to biomechanically relevant anatomy; (b) a concise synthesis of the accumulated biomechanical evidence demonstrating degeneration correlated with age; and (c) appropriate surgical interventions in some age-related spinal diseases.2 A discussion about muscular degeneration has been avoided in the interest of brevity, the overall context, and the focus of the review.
ANATOMY
Normal Adult Anatomy
The vertebral column (spine) comprises the neck and the back. It forms a key part of the axial skeletal system: it protects the spinal cord, supports the weight of the trunk, provides posture, and offers a partially stiff and flexible axis for the body.3 The vertebral column in an adult consists of 33 vertebrae divided into five regions: 7 cervical (C1-C7), 12 thoracic (T1-T12), 5 lumbar (L1-L5), 5 sacral (S1-S5), and 4 coccygeal. The first 24 vertebrae are movable while the rest fuse by late adulthood. Intervertebral disks (IVDs) between vertebrae contribute to the flexibility of the column (Fig. 10.1). However, there is no IVD between the occiput and C1, or between C1 and C2. The vertebral column has four curvatures: cervical, thoracic, lumbar, and sacral. The thoracic and sacral curvatures are concave anteriorly (kyphosis), while the cervical and lumbar curvatures are concave posteriorly (lordosis).4,5 A vertebra consists of a vertebral body, a vertebral (neural) arch protecting the spinal cord, and several processes as sites for muscle attachment (Fig. 10.2). The bony tissue in a vertebra is composed of cancellous and cortical bone. Cancellous bone is a highly porous structure consisting of a network of rod-and-plate-shaped trabeculae surrounding an interconnected pore space that is filled with bone marrow. The main distinguishing factor between cortical and cancellous bone is their porosity: 5% to 20% and 40% to 95% for cortical and cancellous bone, respectively. The vertebral body is the anterior part of a vertebra that supports body weight and transmits ground reaction forces. A hyaline cartilage covers the cranial and caudal ends of the vertebral body. It also provides protection to the vertebral bodies and helps in diffusion of nutrients and waste fluids between the IVD and the vertebral body.6,7 The superior and inferior processes of adjacent vertebrae—known as facets or zygapophysial joints—articulate in a way to constrain motion. Depending on the position and orientation, these joints also bear part of the body weight and provide resistance to motion across the adjacent vertebrae, like slippage.5
IVD is somewhat cylindrical in shape and is made up of three structures: annulus fibrosus (AF), nucleus pulposus (NP), and cartilaginous endplates (CEPs). NP is the central hydrated region of the IVD and develops hydrostatic pressure because of the compressive stresses exerted on it.7 NP is surrounded by laminated AF circumferentially and by CEPs at the cranial and caudal ends. AF and CEPs absorb the hydrostatic pressure developed by the NP and prevent it from protruding into the adjoining areas like the spinal canal. IVD allows bending and
rotation of the spine and helps in the transmission of loads all the while dissipating some of the energy. The ligaments that stabilize the spine are passive structures: anterior longitudinal ligament (ALL), posterior longitudinal ligament (PLL), ligamenta flava (LF), ligamentum nuchae (LN), interspinous ligament (ISL), intertransverse ligament (IL), and capsular ligament (CL). Apart from these, there are additional ligaments present in the upper cervical region.8
rotation of the spine and helps in the transmission of loads all the while dissipating some of the energy. The ligaments that stabilize the spine are passive structures: anterior longitudinal ligament (ALL), posterior longitudinal ligament (PLL), ligamenta flava (LF), ligamentum nuchae (LN), interspinous ligament (ISL), intertransverse ligament (IL), and capsular ligament (CL). Apart from these, there are additional ligaments present in the upper cervical region.8
A functional spinal unit (FSU) consists of two adjacent vertebrae, the intervertebral disk, and all adjoining ligaments between them (Fig. 10.1). It is the smallest physiologic motion segment of the spine to exhibit the key anatomic and biomechanical characteristics similar to those of the entire spine.9
Changes in Anatomy and Disease Manifestation with Age
With age, most of the spinal structures show degenerative changes and some of these are described here.
Trabecular Architecture and Cortical Thickness
In a vertebral body, trabecular bone tends to become more rod-like and its thickness decreases with age. Also, there is an increase in the anisotropy (the resistance to forces is no longer in all directions) of the trabecular structure. This increased anisotropy allows increased axial load-carrying capacity. However, there is a down side: increased vulnerability to fractures due to off-axis impacts.10 Cortical thickness also changes with age and osteoporosis. In an osteoporotic spine, upper thoracic vertebral bodies have 15% thinner ventral cortical bone when compared with control specimens. Lower thoracic and lumbar vertebral bodies also show a 30% decrease in the dorsal cortical thickness compared to control. Even in a normal spine with no signs of osteoporosis, a significant decrease in the cortical thickness below the T8 vertebral body has been found.11
Osteoporosis and Vertebral Compression Fractures
Osteoporosis is a condition of skeletal fragility in which bone strength is reduced to the extent that fractures occur with minimal force, often during routine activities. Primary osteoporosis is classified into two major types: one related to menopausal estrogen loss (type I) and the other to aging (type II). Type I osteoporosis signifies a loss of trabecular bone after menopause in women, while type II osteoporosis suggests a loss of cortical and trabecular bone in both men and women as a result of age-related bone loss. One of the major predictors of osteoporosis is bone mineral density (BMD). On average, BMD reaches its peak at 24 to 25 years of age and decreases thereafter.12, 13, 14
A vertebral compression fracture (VCF) is the collapse of a vertebra due to trauma or a weakened vertebra in a subject with osteoporosis (Fig. 10.1). VCFs are associated with pain and progressive vertebral body collapse resulting in spinal kyphosis. The initial acute pain can be incapacitating and may become chronic in some cases. Chronic pain is a result of incomplete vertebral healing accompanied by progressive vertebral body collapse, pseudoarthrosis at the involved region, or altered spinal kinematics. Prolonged inactivity after a VCF can lead to further bone loss, muscle attrition, and an increased risk of additional fracture.12
Disk Degeneration
IVDs undergo wear and tear with age, which is exacerbated by lower nutrition supply and waste exchange (Fig. 10.3).15,16 Intervertebral disk degeneration (IDD) begins as early as 20 years of age, and autopsies suggest that 97% of individuals over 50 years of age have disk degeneration.17, 18, 19, 20 Manifestation of degeneration begins with decrease in the proteoglycan content of the NP and hence its water content, which results in reduced intradiscal pressure and height. Radial tears, fissures, protrusions, osteophyte formations, facet joint arthritis, and Schmorl nodes (IVD protrusions into the vertebral body) may form along with pain, worsening the degree of degeneration.15,21 Disk degeneration is classified in terms of grades ranging from grades I to V: grade I refers to disk with homogeneous and distinct NP and AF, with normal disk height. On the other extreme, grade V refers to inhomogeneous and indistinct NP and AF, with collapsed disk space.22
Annular Tear and Disk Herniation
Annular tear mostly accompanies IDD, but it could also occur early in the cascade due to strenuous activities (Fig. 10.3). This condition leads to extrusion of disk nucleus into the spinal canal, leading to herniation. Confined herniation occurs from the protrusion of NP, with outermost layer of AF remaining intact.23,24
Spinal Stenosis, Osteoarthritis, and Spondylolisthesis
Facet joints undergo degeneration because of overuse or high loading conditions. Typically, facet degeneration starts with degeneration of cartilage, leading to synovial space inflammation and joint space narrowing, ultimately resulting in osteophyte formation. This may lead to stenosis or spondylolisthesis.25, 26, 27 It has been suggested that disk degeneration precedes facet degeneration.25,26,28 Hyaline cartilage traverses the synovial joint surface of a facet joint and is critical for its proper function. Its loss is a common finding with age. Radiographically, this can be seen as reduction in joint space on magnetic resonance imaging (MRI).
In most cases, this loss is accompanied by a variable amount of inflammatory destruction of the cartilage and synovial lined joint capsule, known as inflammatory pannus. Depending on the severity and duration of the disease, the cartilage may be completely lost and the bare bones may start to rub against each other, leading to eburnation, that is, new bone formation. It is achieved by increasing its surface area through osteophyte formation (Fig. 10.4).
This is called osteoarthritis.29 Spondylolisthesis is the slippage of one vertebra relative to the adjacent vertebra, and it often results from asymmetric degeneration of either the IVD or the facet joints. The imbalance in stresses, due to asymmetric degeneration of these joints, can lead to an asymmetric deformity that further exacerbates the asymmetric loading. This becomes a closed loop cycle that promotes progression of instability. The deformity may occur along
any of the three axes: vertical axis (axial rotation), lateral translation, or anterior translation (Fig. 10.5). The degenerative changes that occur during spondylolisthesis can also lead to spinal stenosis.30,31 Spinal stenosis is an abnormal narrowing of the spinal canal that could occur in any region of the vertebral column. IDD leads to altered local and segmental mechanics, generating compensatory changes like hypertrophy of bone, facets, and ligaments. Along with degenerative changes occurring within the normal aging spine, the surrounding musculature also undergo atrophy with age. Therefore spinal column stability depends on the hypertrophied bone, facet joints, and ligaments. These hypertrophied structures result in the narrowing of the spinal canal or the foramen, which ubiquitously occurs in aging spine (Fig. 10.2).32
any of the three axes: vertical axis (axial rotation), lateral translation, or anterior translation (Fig. 10.5). The degenerative changes that occur during spondylolisthesis can also lead to spinal stenosis.30,31 Spinal stenosis is an abnormal narrowing of the spinal canal that could occur in any region of the vertebral column. IDD leads to altered local and segmental mechanics, generating compensatory changes like hypertrophy of bone, facets, and ligaments. Along with degenerative changes occurring within the normal aging spine, the surrounding musculature also undergo atrophy with age. Therefore spinal column stability depends on the hypertrophied bone, facet joints, and ligaments. These hypertrophied structures result in the narrowing of the spinal canal or the foramen, which ubiquitously occurs in aging spine (Fig. 10.2).32
BIOMECHANICAL CHANGES WITH AGE
Creep Characteristics of Intervertebral Disks
With the application of a sudden load, a nondegenerated disk creeps (i.e., deforms) slowly, as compared to a degenerated disk. This implies that the viscoelastic property (i.e., resistance to deformation and return to original shape) of an IVD attenuates with age as the disk degenerates. This results in less shock absorption and uneven stress distribution (Fig. 10.6).33
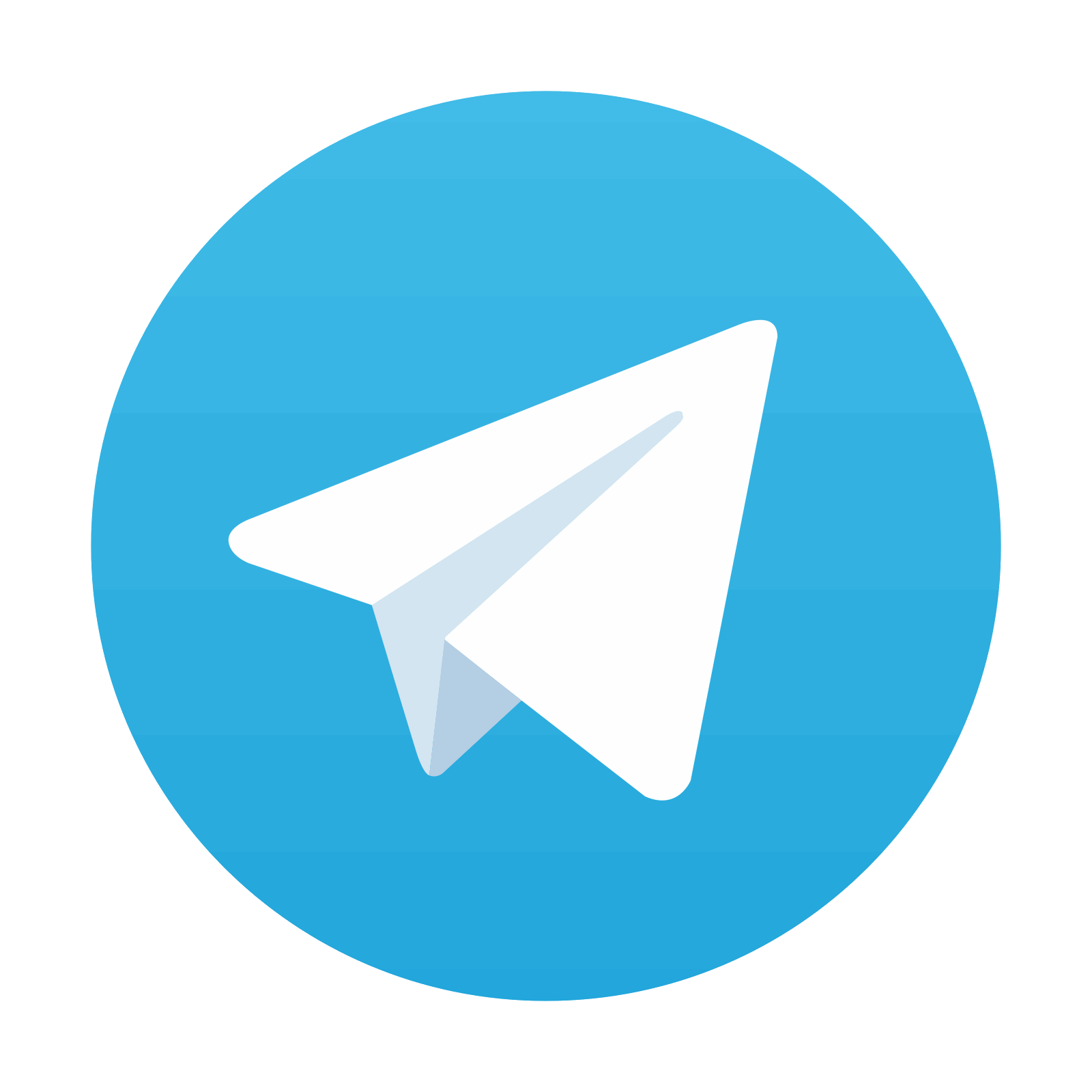
Stay updated, free articles. Join our Telegram channel

Full access? Get Clinical Tree
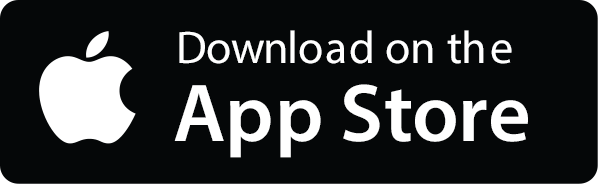
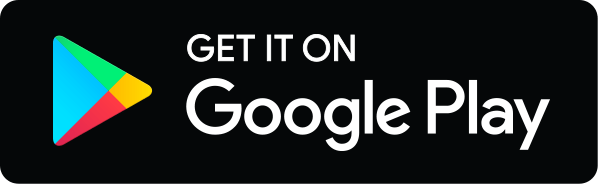