Introduction
The last 10 years has seen the rapid advancement of fracture fixation devices and surgical techniques. The development of preshaped locking plates in concert with minimally invasive surgical exposures has revolutionized the trauma surgeon’s ability to treat complex periarticular injuries. Percutaneous instrumentation, the refinement of insertion portals, and the development of blocking screw techniques has expanded the range of intramedullary (IM) nailing from midshaft injuries to the articular margins. Likewise, the arena of orthobiologics has seen tremendous technological advancements in biomaterials and biologics in an attempt to avoid the perceived morbidity associated with the harvest of autogenous iliac crest bone graft (AICBG). Multiple materials have become available to enhance fracture healing through a myriad of biologic pathways. The purpose of this chapter is to review the basic fund of knowledge on the topic of bone graft substitutes. Currently available adjuvants for approved clinical use will be discussed. As well, the current levels of evidence in supporting the use of these various materials will be reviewed.
Biology of Graft Substitutes
The biology of bone grafts and their substitutes is appreciated from an understanding of the bone formation processes of osteogenesis, osteoinduction, and osteoconduction.
Graft osteogenesis: This is the ability of cellular elements within a donor graft, which survive transplantation, to synthesize new bone at the recipient site. Osteogenesis can occur in two ways. Surface osteoblasts can survive the transplantation by receiving nutrition through diffusion at the recipient site, and then proliferate to form more living bone tissue. There is more surface area in cancellous graft, and thus it has more potential for surviving cells than a cortical graft. Likewise the transplantation of marrow elements alone has demonstrated this ability to survive and form bone.
These transplanted cells or circulating pluripotential cells must have the appropriate substrate to attach to, or become attached to, once the cells have localized to the site of defect or injury. This substrate site for cellular attachment has to have the appropriate three-dimensional architecture to allow for these cells to proliferate. Subsequently, these cells then use this substrate as a scaffolding through which to build bone. This three-dimensional process involves vascular proliferation and ingrowth of capillaries along the open spaces in the substrate and thus the porosity of these materials is critical. Attachment is then followed by the differentiation of cells into bone-forming cells types, and the production and remodeling of bone then proceeds.
Graft osteoconduction: A major category of available graft substitutes for defect management intervention is to provide a lattice-like substrate material that will facilitate the attachment and proliferation of osteoblastic cellular elements.
Graft osteoinduction: As all skeletal tissues evolve from mesenchyme, undifferentiated mesenchymal cells make a genetic commitment to a particular cellular lineage early in the developmental or repair process. In the case of repair, some stimulus must signal the undifferentiated mesenchymal cells to differentiate along a chondro-osteogenic pathway. Prior to this differentiation into an osteogenic lineage, these cells are affected by multiple factors that provide chemotactic and mitogenic stimuli to these cells. This process is jump-started by graft-derived growth factors, such as transforming growth factor- ß, insulin-like growth factors 1 and 2, platelet-derived growth factor, and others. They influence these cells to migrate, attach, and multiply at the locale that provides a competent osteoconductive substrate as a site of cellular attachment. This phenomenon, known as osteoinduction, is defined as “a process that supports the mitogenesis of undifferentiated mesenchymal cells leading to the formation of osteoprogenitor cells with the capacity to form new bone.” Thus, any material that induces this process could be considered to be osteoinductive.
The concept of osteoinductive new bone formation is realized through the active recruitment of host mesenchymal stem cells (MSCs) from the surrounding tissue, which differentiate into bone-forming osteoblasts. This process is facilitated by the presence of growth factors within the graft, principally bone morphogenic proteins.
Marshall R. Urist described the induction principle in the 1960s. The concept of bone matrix—demineralized bone matrix (DBM)—is that this material contains properties that can induce de novo new bone formation when implanted into an extraskeletal site. It should be noted, however, this response is seen in animal models but has not been demonstrated in humans.
Urist and his colleagues soon identified a protein that they named bone morphogenetic protein (BMP). Other molecules were soon identified and helped to characterize an entire family of osteoinductive molecules. This family of specific factors now contains at least 15 BMPs and is part of the larger transforming growth factor-β (TGF-β) superfamily of molecules.
Protein extracts derived from bone can initiate the process that begins with cartilage formation and ends in de novo bone formation. The critical components of this extract, BMPs, which direct cartilage and bone formation as well as the constitutive elements supplied by the animal during this process, have long remained unclear. Amino acid sequence has been derived from a highly purified preparation of BMP from bovine bone. Now, human complementary DNA clones corresponding to three polypeptides present in this BMP preparation have been isolated, and expressions of the recombinant human proteins have been obtained.
Each of the three (BMP-1, BMP-2A, and BMP-3) appears to be independently capable of inducing the formation of cartilage in vivo. Two of the encoded proteins (BMP-2A and BMP-3) are new members of the TGF-β supergene family, while the third, BMP-1, appears to be a novel regulatory molecule. BMP factors can be synthesized by recombinant gene technology or derived from autologous bone, allogeneic bone, or from DBM.
DBM is allogenic bone that has undergone the acid extraction of the mineralized extracellular matrix of the allograft bone. In theory, the noncollagenous proteins, including osteoinductive proteins such as the BMPs, remain viable while the structural portion of the allograft has been removed.
All bone graft and bone-graft-substitute materials can be described through these three processes. While fresh autologous graft has the capability of supporting new bone growth by all three mechanisms, it may not be necessary for a bone graft replacement material to have all three properties inherent in that material in order to be clinically efficacious ( Table 4-1 ). When inductive molecules are locally delivered on a scaffold, ultimately, MSCs are attracted to that site and are capable of reproducibly inducing new bone formation provided minimal concentration and dose thresholds are met. In some clinical studies, osteoinductive agents have been shown to potentially perform superiorly compared to conductive materials alone.
Osteoconductive Scaffold | Osteoinductive Growth Factors | Osteogenic Living Cells | |
---|---|---|---|
Synthetic Ca + ceramics | • | ||
Marrow concentrates | • | ||
Bone morphogenic protein (BMP) | • | ||
Autograft | • | • | • |
Platelet-rich concentrates | Osteopromotive indirect cellular effect No intracellular transcription | ||
Banked demineralized bone matrix (DBM) | • | • |
When bone marrow aspirate (viable stem cells) is applied to an osteoconductive scaffold, these cells are still reliant on the local mechanical and biological inductive signals to ultimately form bone. Similarly, osteoconductive materials work well when filling noncritical size defects that would normally heal easily without any additional adjuvants added. However, in more challenging critical-size defects, all three types of these materials are necessary as an adjunct in order to achieve efficacy equivalent to autograft.
Stages of Bone Graft Incorporation
Bone grafts and bone graft substitutes incorporate via a well-defined pathway that can be divided into five distinct stages of host response, with the duration of each phase depending on the type of graft or adjuvant material used.
Stage 1: Initial injury and hemorrhage: Initiates the pathway with the degranulation of platelets at the site of injury.
Stage 2: Inflammation: Under the influence of many active cytokines that are produced at the site of injury. These cause migration of cells to the site of injury.
Stage 3: Vascular proliferation and ingrowth. Under the influence of many cytokines invading capillaries bring perivascular tissue with mesenchymal cells that can differentiate into osteoprogenitor cell lines. A competent conductive substrate is required for this process to occur. This vascular invasion can be significantly inhibited by nonsteroidal antiinflammatory medications, which will inhibit this process and thus alter the fracture healing pathway.
Stage 4: The fourth stage consists of osteoclastic resorption of the avascular (dead) bone graft lamellae and simultaneous production of new bone matrix by osteoblasts.
Stage 5: In the final stage, the newly formed bone is remodeled and reoriented based on the mechanical environment of the host site.
Orthobiologic interventions have been specifically designed to target these stages to achieve a specific effect. Each broad category of intervention will be discussed in terms of the desired effect these material have on the specific stage of graft incorporation. The gold standard and prime material that all others are compared to is AICBG.
Autogenous Iliac Crest Bone Graft
Fresh cancellous autograft provides the quickest and most reliable type of bone graft. Its open structure allows rapid revascularization; a 5-mm graft may be totally revascularized in 20 to 25 days. These grafts depend on ingrowth of host vessels and perform best in well-vascularized beds. The large surface area of harvested autograft allows for survival of numerous graft cells. It is estimated that approximately 30 mL of graft can be reliably harvested from an anterior iliac crest. However, many other sites of harvest have been described, differing only in the amount of graft obtained from each harvest site (see Table 4-1 ). Recent literature has demonstrated histologic differences between iliac crest and tibial bone graft, suggesting superiority of iliac crest in terms of osteogenic and hematopoietic progenitor cell content. Studies document success rates approaching 100% for subcritical-size defects (1- to 2-cm defects) requiring 20 mL or less of autograft.
There are many issues regarding AICBG because of limited quantity available, and the reported rates of postoperative pain from the graft harvest site. Substantial rates of complications related to the harvest site have been reported. It has been thought that this technique is restricted to short defects, in the range of 4 to 6 cm. Numerous studies report favorable union results for critical-size defects up to 4 cm. However, in many of these studies, multiple graft procedures were required to achieve solid union.
The ability to obtain substantial amounts of autogenous graft material would appear as an advantage for the treatment of critical-sized defects. The reamer-irrigator-aspirator (RIA; Synthes, Paoli, PA) offers a technique to achieve substantial amounts of graft volumes for the treatment of larger segmental defects. The medullary canal of the femur or tibia is reamed with a device designed to collect the reamings and deliver them for potential grafting procedures. Variable amounts of harvested graft with this technique have been reported in the literature and range from 30 to 90 mL. A recent comparison between a historical control group using anterior iliac harvesting (40 patients) versus a study group using femoral shaft RIA harvesting (41 patients) documented on average 25 to 75 mL of harvested RIA graft (average = 40.3 mL). The authors reported a favorable union rate with RIA bone grafting (37 of 41 patients) versus AICBG (32 of 40 patients), although not statistically significant. There were significantly lower postoperative harvest site pain scores from the RIA group versus the AICBG group at 48 hours, 48 hours to 3 months, and greater than 3 months ( P = 0.001, 0.001, and 0.004, respectively). There were a total of two complications related to the graft harvest site in the RIA group (one perforation of the distal anterior femoral cortex treated conservatively and one excessive reaming of the femoral neck treated with prophylactic cannulated screws) versus 12 harvest site complications in the AICBG group (3 infections, 1 hematoma, and 8 patients with numbness).
This study has several limitations including the concurrent use of BMP-2 in most cases. This somewhat limits the ability to draw strong conclusions regarding the relative efficacy of RIA bone graft versus AICBG from this study.
A recent study reported on the treatment of 20 bone defects ranging from 2 to 14.5 cm (average = 6.6 cm) using RIA bone graft. Eighteen of the 20 patients were initially treated with an antibiotic cement spacer using the Masquelet technique ( Fig. 4-1 ). The average graft volume obtained using the RIA was 64 mL. Seventeen of 20 bone defects ultimately healed, although 7 of these required repeat surgery. The authors reported no significant complications related to the bone graft harvest site (see Fig. 4-1 ).

Numerous basic science studies have demonstrated the biologic potential of RIA bone graft. Investigators have documented elevated amounts of osteoinductive growth factors and osteoprogenitor and endothelial progenitor cell types compared to AICBG. The RIA filtrate contains large numbers of MSCs that could potentially be extracted without enzymatic digestion and used for bone repair without prior cell expansion. Medullary autograft cells harvested using RIA are viable and osteogenic. Cell viability and osteogenic potential were similar between bone grafts obtained from both the RIA system and the iliac crest.
Elevated levels of fibroblast growth factor-α (FGF-α), platelet-derived growth factor (PDGF), insulinlike growth factor-1 (IGF-1), TGF-β 1 , and BMP-2 were measured in the reaming debris as compared to iliac crest curetting’s. However, vascular endothelial growth factor (VEGF) and FGF-β were significantly lower in the reaming debris than from iliac crest samples. In comparing platelet-rich plasma (PRP) and platelet-poor plasma (PPP), all detectable growth factors, except IGF-1, were enhanced in the PRP. In the reaming irrigation FGF-α (no measurable value in the PRP) and FGF-β were higher, but VEGF, PDGF, IGF-1, TGF-β 1 , and BMP-2 were lower compared to PRP. BMP-4 was not measurable in any sample. The bony reaming debris is a rich source of growth factors with a content comparable to that from iliac crest. The irrigation fluid from the reaming also contains growth factors. Although the early evidence regarding RIA bone grafting is encouraging, there is currently a lack of high-level comparative evidence.
Osteoconductive Graft Substitutes
There is considerable interest in creating osteoconductive matrices using nonbiological porous structures implanted into or adjacent to bone. The host substrate must mimic the cancellous bony architecture and have very specific surface kinetics to facilitate the migration, attachment, and proliferation of MSCs, which then differentiate into osteoprogenitor cells (augmentation of stages 2 and 3 of graft incorporation). Broad categories of these materials are available and, in general, are classified as calcium ceramics. These include the specific materials of calcium sulfate, calcium phosphate, synthetic tricalcium phosphate as well as β-tricalcium phosphate, and coralline hydroxyapatite (HA).
The history of bioceramics dates back to 1892 with the use of calcium sulfate for space-occupying lesions. Calcium sulfate has the distinction of being the alternative that is both one of the simplest as well as that which has the longest clinical history as a synthetic bone graft material—spanning more than 100 years.
The original material was plaster of Paris, which is noninflammatory and nonreactive and encouraged bone healing in a contained lesion. Peltier took commercial-grade plaster of Paris, which was then mixed with water, poured into molds made of wax paper or aluminum foil, and then allowed to set, forming small pellets or columns. He performed a series of bone defect studies in dogs to determine the role these materials had in the ability to heal these defects. From his experiments, he determined that the plaster of Paris itself does not stimulate osteogenesis. Its chief effect was found to be a mechanical one of preventing the collapse of the periosteal tube and favoring regeneration. In this way the material provided a supportive scaffolding. There is no doubt, however, that subperiosteal resections in which plaster of Paris columns were inserted regenerated, in whole or in part, more frequently than was the case in subperiosteal resections alone.
Subsequently, coralline HA was reported for similar lesions followed by other ceramics. All of these bone graft substitutes have the advantage of being nonimmunogenic, noninflammatory, in an unlimited supply, and packaged sterile.
Calcium Sulfate Substitutes
Calcium sulfate was one of the first orthobiologic materials to be used commercially as a bone graft substitute. Calcium sulfate has a crystalline-independent rate of incorporation and is very consistent in terms of dissolution and incorporation. The crystalline structure is consistent throughout a whole range of materials, with a constant rate of osteointegration. In contrast to calcium phosphate, calcium sulfate behaves as a true salt, that is, if it egresses into the joint, it quickly dissolves into sulfate and calcium ions and is then absorbed into the synovium. This resorption mechanism involves a fluid exchange mechanism and may promote excessive drainage if used in wounds with questionable soft tissue coverage or integrity. A low but consistent complication rate, specifically serous drainage from the wound as the calcium sulfate absorbs, has been reported. This complication is higher when the material is used in higher volumes (greater than 20 mL) or in subcutaneous bones (tibia, ulna).
This may cause a potential increase in osmotic load at the site of implantation. Therefore, to avoid subsequent drainage at the graft site, the calcium sulfate product should be reserved for situations with adequate blood supply and competent soft tissue coverage. Additionally, this material should be used in a contained defect only. Studies document that implanted calcium sulfate pellets in contact with joint synovial fluid are at risk for resorption without any significant bony healing response. If calcium sulfate pellets are to be implanted in periarticular locations, complete bony containment is necessary.
Calcium sulfate hemihydrate has been used for many years as a self-setting biomaterial due to its good setting properties. The fairly rapid degradation rate of these materials, which occurs in 3 to 4 months, was once viewed as an advantage. However, as these materials began to be used to support articular subchondral surfaces in cases of periarticular plateau and pilon fractures, this rapid degradation becomes a distinct disadvantage. Transition to full weight bearing occurs normally at 3 to 4 months after surgery, and many cases of late articular collapse have been subsequently reported due to this rapid incorporation with the simultaneous loss of articular support. Additionally, this material demonstrates rapid loss in its mechanical compressive strength following implantation, when compared to the phosphate ceramics.
This combination of rapid degradation rate, speedy loss of compressive strength, and lack of bioactivity have currently limited its application for bone defect management. Three case series examining the use of calcium sulfate for the treatment of bone nonunion revealed a significant failure rate, suggesting that this material, used in isolation, is not optimal to promote union in that setting.
The current best use of this material appears to be that of a carrier for adjuvant antibiotics as a treatment for osteomyelitis. The characteristics regarding rapid resorption and degradation are now advantageous for delivering high-dose antibiotics. McKee and colleagues demonstrated results for the treatment of chronic osteomyelitis and infected nonunions, using an antibiotic-impregnated calcium sulfate pellet. He felt that this was equivalent to standard surgical therapy in eradicating infection and reducing the number of subsequent surgical procedures necessary.
There is level I and II evidence (one randomized trial, one case-control study, one prospective cohort study) that antibiotic-impregnated bioabsorbable calcium sulphate has the potential to reduce the number of procedures and surgical morbidity associated with the surgical treatment of chronic osteomyelitis and infected nonunion while maintaining a high rate of infection eradication. Calcium sulphate remains an inexpensive, safe, reliable bone-void filler that can also serve as an absorbable delivery vehicle for antibiotics or other compounds.
Calcium Phospate Substitutes
Calcium phosphate substitutes are osteoconductive, but they are not osteoinductive unless growth factors, BMPs, or other osteoinductive substances are added to create a composite graft. Calcium phosphate is available in a variety of forms and products, including ceramics, powders, and cements. Ceramics are highly crystalline structures created by heating nonmetallic mineral salts at temperatures greater than 1000° C, a process known as sintering . These phosphate materials have variable rates of osteointegration based on their crystalline size and stoichiometry.
Hydroxyapatite
Synthetic HA is a crystalline calcium phosphate osteoconductive bone substitute that is also manufactured as a ceramic through a sintering process. This material was some of the first available to be used clinically as a bone graft substitute. Porous HA (Interpore 500) was formed by conversion of the Porites goniopora coral exoskeleton, with pores averaging 600 µm and pore interconnections averaging 260 µm in diameter.
Clinical studies with this material used for augmentation of tibial plateau fractures were successful when used with internal fixation. No significant radiographic or clinical differences were appreciated between those patients that were randomized to have the defect filled with either autogenous bone or porous HA.
The investigators felt that a major drawback of the material was that the rate of incorporation was very slow. The appositional process of incorporation of the implant was confirmed by the finding that only 66.5% of the surface of the Interpore 500 was covered with bone ingrowth at 12 months. Thus, a relatively long incorporation time was documented.
Other investigators studied the ability of this material to act as a conductive substrate and determine the effectiveness of coralline HA as a bone graft substitute for lumbar spine fusion when used in combination with bone marrow, or when mixed with autogenous bone graft (ABG), or combined with an osteoinductive material (BMP). The data indicated that coralline HA with bone marrow was not an acceptable bone graft substitute used alone for posterolateral spine fusion when compared to autograft.
When combined with AICBG, coralline HA served as a graft extender yielding results comparable to those obtained with autograft alone. In addition, coralline HA served as an excellent carrier for the bovine osteoinductive bone protein extract yielding superior results to those obtained with autograft or bone marrow. As with other characteristics of these materials, the rates of osteoinduction were greatest when a porous architecture was maintained. Animal studies have suggested that HA may have some osteoinductive properties in addition to its osteoconductive capabilities.
The crystalline structure dictates the rate of osteointegration. These materials integrate via a cell-mediated response, and the pore structure found in these materials serves as sites for cellular attachment. This porosity makes these materials very brittle with minimal tensile strength. Because of these material properties, and concerns regarding very slow bone formation, HA used alone is not commonly used as an osteoconductive bone substitute at this time.
The porosity of these materials is the primary factor in determining the ability to foster ingrowth and osteointegration. No osseous ingrowth occurs with pore sizes of 15 to 40 µm. Osteoid formation requires minimum pore sizes of 100 µm, with pore sizes of 300 to 500 µm reported to be ideal for osseous ingrowth. Some authors, however, have reported that pore size may be less critical than the presence of interconnecting pores for osseous ingrowth. Interconnecting pores prevent the formation of blind alleys, which are associated with low oxygen tension; low oxygen tension prevents osteoprogenitor cells from differentiating into osteoblasts.
Highly porous interconnected materials have abundant sites available for cellular interactions, which help these materials osteointegrate faster. This is accompanied by a corresponding decrease in the compressive strength afforded. If the material is designed with minimal porosity, the rate of osteointegration will be prolonged because of the paucity of cellular interactions. The corresponding compressive strength will also be very high. As noted, their ability to provide structural support is dependent on the degree of porosity inherent in each unique material that can be highly manipulated. These materials have the advantage of incorporating at a slower rate than calcium sulfate materials. They increase bone formation by providing an osteoconductive matrix for host osteogenic cells to create bone under the influence of host osteoinductive factors shores.
Tricalcium Phosphate
Tricalcium phosphate (TCP) is a commonly available resorbable ceramic. It can be obtained in block, granular, powder, or putty form. Coralline ceramics are formed by thermochemically treating coral with ammonium phosphate, leaving TCP with a structure and porosity that is similar to that of cancellous bone.
TCP is less brittle and has a faster resorption rate than HA because of the increased porosity. Animal studies demonstrated that 95% of calcium phosphate is resorbed in 26 to 86 weeks. This is much faster when compared to the previous HA reported rates of osteointegration.
TCP and HA have been combined into a biphasic calcium phosphate composite that has a faster resorption rate than pure HA. In a clinical study using a composite graft, a mixture of porous beads composed of 60% HA and 40% TCP ceramic and fibrillar collagen was used as a graft substitute and combined with autogenous bone marrow. Collagraft (Zimmer and Collagen Corporation) was randomized against cancellous iliac crest autografts in the treatment of long bone fractures. This material appeared to function as well as autogenous graft when used in the treatment of acute long bone fractures.
Calcium phosphate can also be manufactured as cement, by adding an aqueous solution to dissolve the calcium, which is followed by a precipitation reaction in which the calcium phosphate crystals grow and the cement hardens. The primary advantage of cements over blocks, granules, or powders is the ability to custom-fill defects and produce increased compressive strength. However, cement can be extruded beyond the boundaries of the fracture, potentially damaging the surrounding tissue. This is especially problematic if these materials extrude into a joint cavity following repair of a subchondral defect such as a tibial plateau. This presents a potential disadvantage of these phosphate materials, as they will not dissolve if they happen to migrate into the joint. The ability of calcium phosphate bone substitutes to act as a bone-void filler has been documented in multiple preclinical animal studies and biomechanical and human case series.
One such representative biomechanical study evaluated the fatigue strength of calcium phosphate–augmented repairs versus ABG repairs for lateral tibia plateau fractures. Reproducible split-depression fractures were simulated and repaired with each specimen randomly assigned to either calcium phosphate or ABG as augmentation. Calcium phosphate–augmented repairs subsided less and were more stiff during the fatigue loading than were ABG repairs at the 70,000, 140,000, and 210,000 cycle intervals ( P < 0.03). The authors concluded that the calcium phosphate repairs had significantly higher fatigue strength and ultimate load than ABG repairs and may serve to increase the immediate weight-bearing capabilities of the repaired plateaus in clinical practice.
A large clinical retrospective case series reviewed 43 patients with traumatic bone defects or nonunions treated. Defects of the femur, tibia, calcaneus, humerus, ulna, or radius had treatment augmented with TCP. Ninety percent of the fractures and 85% of the nonunions had united at the time of follow-up, average of 12 months. The authors concluded that TCP was a useful substitute for cancellous bone if the local biology provided a suitable blood supply. This was deemed necessary to provide competent cells and circulating inductive factors.
The use of injectable bone calcium phosphate cements offers the opportunity to support the reduced joint surface without open bone grafting. This is a valuable adjuvant, as less invasive fixation approaches are becoming widely accepted and the ability to limit the exposures for grafting and subchondral defect augmentation would also be a valuable tool. Jubel and colleagues evaluated the clinical and radiologic outcomes of an injectable calcium phosphate material (Norian SRS) used for tibial plateau fractures. The time interval from surgery to partial weight bearing was 3.7 weeks. The results demonstrated that this material can be successfully used to fill metaphyseal bone defects in tibial plateau fractures. The clinical and radiologic results were comparable to those of fractures treated with autologous bone graft. The high compression strength allows early full weight bearing without the risk of secondary loss of reduction. Soft tissue reactions due to the cement were not observed. However, on all radiographs taken 36 months after the operation, the phosphate cement block was still visible indicating a very slow rate of osteointegration, which may be of some concern to some clinicians. Once the transition to full weight bearing has been achieved, it would be desirable to have the material almost completely integrated. There are newer phosphate materials now available that have shorter times to complete osteointegration as these crystalline structures can be manipulated accordingly.
In a critical study by Russell and colleagues, the efficacy of a bioresorbable calcium phosphate cement was compared with standard autogenous iliac bone grafting in a multicenter, prospective, randomized study for the treatment of these osseous defects. Randomization to treatment with calcium phosphate cement (82 fractures) or autogenous iliac bone graft (38 fractures) occurred at the time of surgery. There was a significantly ( P = 0.009) higher rate of articular subsidence during the 3- to 12-month follow-up period in the bone graft group. The bioresorbable calcium phosphate cement used in this study appeared to be a better choice, at least in terms of the prevention of subsidence, than autogenous iliac bone graft for the treatment of subarticular defects associated with unstable tibial plateau fractures.
Many studies have specifically evaluated these materials as bone graft substitutes in the management of subchondral bone defects associated with tibial plateau fractures. A meta-analysis study compared calcium phosphate cement substitutes directly to these other conductive substrate materials used for plateau augmentation: HA granules, calcium sulphate, bioactive glass, TCP, DBM, allografts, autografts, and xenografts. Fracture healing was uneventful in more than 90% of the cases over the variable time period of the meta-analysis. Secondary collapse of the knee joint surface ≥2 mm was highest in the biological substitutes group, 8.6% (allograft, DBM, autograft, and xenograft). This is similar to the results noted by Russell in his randomized study comparing autologous iliac bone graft (AIBG) to calcium phosphate cement. Late collapse was less in the HA-treated group, 5.4%, and the material that had the least subsidence was the calcium phosphate cement group. 3.7%. The group that experienced the highest rate of subsidence was the calcium sulfate cases, 11.1%. This is consistent with the rapid dissolution time and relative biomechanical properties of this material discussed previously in this chapter.
The recorded incidence of primary surgical site and donor site infection (3.6%) was not statistically significantly different among all the grafting groups. However, donor site-related pain was reported up to 12 months following AIBG harvest. Shorter total operative time, greater tolerance of early weight bearing, and improved early functional outcomes within the first year after surgery were noted in the studies reporting on the use of injectable calcium phosphate cements. Despite a lack of good quality randomized controlled trials, there is arguably sufficient evidence supporting the use of bone graft substitutes in the clinical setting of depressed plateau fractures.
These materials have also been extensively used for the augmentation of distal radius fractures. Zimmermann and colleagues evaluated patients treated with cement augmentation 2 years after the surgery. The authors concluded that the use of injectable calcium phosphate cement (Norian SRS) to supplement pin and screw fixation was effective in maintaining the reduction of unstable intraarticular distal radius fractures in osteoporotic patients and provided superior functional outcomes at 2 years after the surgery compared to percutaneous pinning alone. With the widespread use of locked plating for these injuries, the efficacy of these materials for use in this situation must be questioned. A recent randomized study sought to determine whether augmentation of volar locking-plate fixation with calcium phosphate bone cement had any benefit over volar locking-plate fixation alone in an elderly patient population with unstable distal radial fractures. The two groups were comparable with regard to age, sex, fracture type, injury mechanism, and bone mineral density. No significant differences were observed between the groups with regard to the clinical outcomes at the 3- or 12-month follow-up examination. No significant intergroup differences in radiographic outcomes were observed at 1-year follow-up.
The authors concluded that augmentation of metaphyseal defects with calcium phosphate bone cement after volar locking-plate fixation offered no benefit over volar locking-plate fixation alone in elderly patients with an unstable distal radial fracture. This highlights the point that with the improved biomechanics that locked plating provides, prospective studies are required to determine the role of these conductive substrates when they are combined with locked plating techniques.
The treatment of intraarticular calcaneal fractures is commonplace in busy trauma practices. Although open reduction and fixation are favored by many authors, increased risk of soft tissue complications makes this method of treatment a challenge. Again the value of an injectable, minimally invasive material to augment posterior facet elevation would be valuable. Wee and colleagues documented in a recent clinical study the safe use of an injectable calcium phosphate cement to augment standard plate fixation of calcaneal fractures. A total of 10 patients with 12 displaced intraarticular calcaneal fractures underwent this method of treatment. Full weight bearing was started at 1 month postoperatively, and no cases demonstrated loss of reduction. The authors felt that the early results with stabilization and augmentation were encouraging.
In a similar study Eisner and colleagues compared patients treated surgically with and without injectable carbonated apatite cement. In the cement group, full weight bearing on the affected extremity was regained at an average of 4 weeks postoperatively. During the study period of 3 years, only a slight decrease in the density of the peripheral zones of the cement block was observed (again referring to the relatively long integration time with these first- and second-generation materials). Complete resorption and remodeling of the bone cement were not complete at 3 years.
These findings should be temporized by the results of an earlier study by Schildhauer and colleagues, who reported on a series of 36 joint depression–type calcaneal fractures treated by internal fixation augmented with calcium phosphate cement. Patients began to bear weight as early as 3 weeks after the surgery without loss of reduction, and no significant difference in functional outcome scores between patients with early versus late weight bearing. Of concern, however, was a finding of an 11% infection rate. The majority of infections occurred in smokers. In spite of the infection rate, the authors concluded that cement augmentation of the fixation for joint-depression calcaneal fractures allowed earlier weight bearing with no change in postoperative outcomes.
Proximal humeral fracture augmentation has also been reported. Reinforcement with calcium phosphate cement in the treatment of proximal humeral fractures with locked plates decreased fracture settling and significantly decreased intraarticular screw penetration and helped to prevent varus deformation in clinical series. Augmentation was able to decrease the tendency for subsequent superior screw cutout from the humeral in biomechanical studies.
Civinini and colleagues reported on the results of the treatment in patients with early-stage osteonecrosis of the femoral head (ONFH) that underwent core decompression, injection of autologous bone marrow concentrate, and the use of a composite injectable bone substitute calcium sulfate and tricalcium phosphate as a mechanical supplementation associated with decompression.
At final follow-up, the mean hip scores increased from 68 points preoperatively to 86 points postoperatively, and radiographic improvement occurred in 29 hips (78.4%). The overall clinical success rate of the procedure was 86.5%. The authors felt that backfilling the defect with an injectable bioceramic for the treatment of early stages of ONFH helped to relieve hip pain and prevent the progression of ONFH in the majority of the cases.
Rouvillain reported on the clinical, radiologic, and histologic findings following high tibial valgus osteotomy (HTVO) augmenting the distraction osteotomy with a micro-macroporous biphasic calcium phosphate wedge. This is one of the first studies to examine the results by histologic analysis of the graft incorporation.
Forty-three knees underwent clinical and radiologic follow-up at days 1, 90, and 365 to evaluate consolidation and bone substitute interfaces. Biopsies were obtained at least 1 year after implantation from 10 patients who requested plate removal. Radiographic consolidation was observed in 98% of patients with no late collapse noted. Histology confirmed normal bony architecture with trabecular and/or dense lamellar bone growth throughout the wedge implants. Radiographs and microcomputed tomography (CT) scan revealed a well-organized, mineralized structure in the newly formed bone. This study confirmed that using medial biphasic calcium phosphate (MBCP) wedges in combination with locked plates offered a simple, safe, and fast surgical technique for HTVO.
Early results have demonstrated that augmentation of femoral neck and intertrochanteric hip fractures with calcium phosphate cement is feasible, with no substantial increase in complications.
A recent meta-analysis was undertaken to evaluate this concept of hip fracture augmentation and came to differing conclusions: 411 studies were identified, of which 22 met the rigorous inclusion criteria, comprising 12 experimental and 10 clinical reports. The clinical studies were evaluated with regard to their levels of evidence. Only four were prospective and randomized. Polymethylmethacrylate (PMMA) and calcium phosphate cements increased the primary stability of the implant-bone construct in all experimental and clinical studies. In randomized, controlled studies, augmentation of intracapsular fractures of the neck of the femur with calcium-phosphate cement was associated with poor long-term results. There was a lack of data on the long-term outcome for trochanteric fractures. Because there were only a few, randomized, controlled studies, there is currently poor evidence for the use of any orthobiologic bone cement in the treatment of fractures of the hip and it should not be undertaken.
Investigators continue to manipulate these calcium phosphate substrates in an attempt to find the optimal resorption/incorporation rate with the optimal strength ratios maintained. No authors of human studies have been able to clearly demonstrate the actual resorption rate of calcium phosphate cement ( Figs. 4-2 and 4-3 ). However, the creation of macropores can significantly improve the resorption rate of these materials. This increased degradation is associated with almost complete bone replacement. Animal studies have shown that up to 80% of the cement is resorbed at 10 weeks, with resorption and replacement with bone continuing for as long as 30 weeks. This process occurs by dissolution as well as by osteoclast resorption. Biomaterials depicted a significant increase in bone content, when compared to ABG, concerning bone regeneration at the same time period.


The lack of osteoprogenitor cells and osteoinductive potential of calcium-based bone substitutes has led to the development of composite grafts in an attempt to accelerate bone formation. A composite graft is created by adding an osteoinductive factor to an osteoconductive calcium phosphate matrix to theoretically increase bone formation. Many clinical series use composite ceramic grafts combining the scaffolding properties of TCP materials with biological elements to stimulate cell proliferation and differentiation.
As previously mentioned, the incorporation times of some of these materials can be prolonged because of a number of factors. In an attempt to improve the incorporation and integration characteristics, investigators combined ultraporous β-TCP synthetic graft material (Vitoss Bone Graft Substitute, Orthovita) with bone marrow aspirates, with the hypothesis that bone marrow aspirate speeds incorporation of the bone graft substitute. The study prospectively examined healing of cavitary defects filled with TCP versus TCP and bone marrow (BM) aspirate (TCP/BM). While significant improvements in radiographic parameters were observed in both TCP groups over 2 years of follow-up, the addition of BM was not found to provide any significant benefit. This highlights the need for comparative data to determine the effectiveness of just randomly combining these adjuvants.
In an effort to improve the performance of calcium phosphate materials, many investigators are manipulating the materials themselves in an attempt to improve the incorporation characteristics or the osteoinductive capabilities of these materials. The inherent osteoinductivity of silicate-substituted calcium phosphate materials was investigated in a sheep muscle pouch study.
Silicate substitution had a significant effect on the formation of bone both within the implant and on the implant surface during the study period. The formation of bone within muscle during the 12-week period showed both silicate-substituted calcium phosphate and stoichiometric calcium phosphate to be osteoinductive in this model. Silicate substitution significantly increased the amount of bone that formed and the amount of bone attached to the implant surface. New bone formation occurred through an intramembranous process within the implant structure. This material manipulation continues to be the focus of great research interest at this time in attempt to develop materials that have both inductive and conductive properties within the same material.
Demineralized Bone Matrix
DBM is formed by acid extraction of the mineralized extracellular matrix of allograft bone. It contains type I collagen, noncollagenous proteins, and osteoinductive growth factors, including BMPs and other inductive factors found in the TGF-β group of proteins. As noted earlier, the TGF-β superfamily includes a number of factors in addition to BMPs. The factors that are known to be osteoinductive are BMPs, growth differentiation factors (GDFs), and possibly TGF-β 1 , TGF-β 2 , and TGF-β 3 . DBM is highly osteoconductive because of its particulate nature and presents a large surface area and three-dimensional architecture to serve as a site of cellular attachment. Thus, when DBM is implanted in an animal, all of these factors potentially work in combination to produce the observed osteogenic response.
DBM is, strictly speaking, allogeneic bone tissue. In theory, the noncollagenous proteins, including osteoinductive proteins such as the BMPs remain viable. However, because the true test of osteoinductivity is whether a material that has been implanted in a nonosseous site forms bone, the inability of allograft bone to do this in human patients argues against allograft bone having substantial osteoinductive activity. DBM has been shown to produce this effect in animal studies, but it too has never demonstrated this effect in human patients. The relative quantities of BMPs in DBMs are low, in the order of 1 × 10 -9 g of BMP per gram of DBM. The osteoinductive variability has been found not only across different DBM products but also among production lots from the same DBM formulation. Bae and colleagues demonstrated that there is higher variability in the concentration of BMPs among different lots of the same DBM formulation than among different DBM formulations. Each individual lot has a different osteoinductive capacity that can vary considerably among different donors. This variability questions the reliability of DBM products and, possibly, their efficacy in providing consistent osteoinduction.
Prior investigations have shown that the osteoinductive potential can vary widely, with influence from both donor and processing sources. There appears to be sex-related differences in donor DBM. DBM derived from female donors appears to have significantly greater concentrations of BMP-2 and BMP-7 than that derived from male donors ( P = 0.0257 and 0.0245, respectively). There was no significant correlation between donor age and the levels of any of the measured BMPs in a study evaluating BMP levels in commercially available DBM preparations. The presence of other growth factors found in DBM has also been evaluated. Wildemann and colleagues attempted to quantify the activity and presence of eight growth factors important for bone healing in multiple different “off-the-shelf” DBM formulations, which are already in human use. Differences between the products were seen in total protein content and the absolute growth factor values. The type of the growth factors found in these preparations was almost comparable between the materials. FGF and BMP-4 were not detectable in any analyzed sample. BMP-2 revealed the highest concentration extractable from the samples without a significant difference between the three DBM formulations.
In general, all the materials had variable concentrations of TGF-β 1 , FGF-α, IGF-1, and PDGF. No differences were accessed for VEGF. This and other similar studies highlight the differences in the growth factor concentrations between the individual materials, independent of the product formulation. As well, there is evidence of differential potencies of each available growth factor within individual DBM preparations, based on the individual manufacturer and manufacturing process.
There are numerous DBM formulations based on refinements of the manufacturing process. They are available as freeze-dried powder, granules, gel, putty, or strips. They have also been developed as combination products with other materials such as allogeneic bone chips and calcium sulfate granules. There is now evidence of differential potencies of DBM preparations based on the manufacturer and manufacturing process. Sterile processing of the bone may also affect the protein effectiveness of these materials. Some tissue banks harvest and process the DBM under “sterile” conditions whereas other manufacturers sterilize the DBM after processing. Gamma irradiation is frequently used to sterilize implanted devices but has limitations when used on biologically active materials and composites.
Studies indicate that if the DBM is irradiated prior to the addition of any aqueous carrier, the activity of DBM in the dry state remains relatively stable with only a small loss of activity. Composites of DBM with a carrier such as lecithin, to which no water has been added, lose activity at approximately the same rate as DBM in the dry form. In composites that contain water, the loss of activity occurs even at much lower levels of radiation exposure. Osteoinductivity of DBM decreased with the increase of gamma-irradiation dose at ambient temperature, whereas no decrease occurred when treated with gamma irradiation at low temperature. However, the hydrated DBM showed diminishing osteoinductivity after 6-month storage at ambient conditions, whereas the DBMs in dry form retained their osteoinductivity after the 6-month storage. The findings in this study indicate that DBM and demineralized bone matrix/acellular dermal matrix (DBM/AM) composites could retain their osteoinductivity when they are in dry configuration and are irradiated at low temperature (−40° C to −70° C).
Gamma irradiation does not change cell attachment to the DBM matrix but has an influence on both stem cell and osteoprecursor cell proliferation rates. Because of the limitations imposed by radiation, it seems most practical to handle DBM aseptically throughout the procedures of compositing pastes, putties, or suspensions, and only if necessary, exposing bone components to radiation sterilization prior to mixing.
In addition, many of the proteins responsible for “turning on” the differentiation process for stem cells will not function if damaged, and protein preservation is therefore important in the delivery of DBM and BMPs. Some DBM products are lyophilized and mixed with carrier at the time of implantation, while others are prehydrated or premixed and could potentially remain on the shelf for a long period of time. If precise control of conditions is not maintained, many proteins might be susceptible to chemical and physical degradation.
Ethylene oxide as a means for sterilization of DBM is used by some manufacturers and has been shown to attenuate its osteoinductive potential. Exposure of DBM to ethylene oxide for the duration required to kill most common bacterial pathogens results in a marked reduction of its osteoinductivity most likely due to destruction of BMPs and other inductive factors. The effect of ethylene oxide has been shown to be dose dependent.
It is clear that processing and sterilization techniques have significant effects on DBM viability. With this in mind, clinicians should be aware of how these factors influence the efficacy of each particular DBM product that they may choose to use in each clinical application.
The shelf life of the product may also vary with the specific carrier, which may or may not affect the overall activity of the product. All available in vitro assays evaluate the DBM without the carrier added by the manufacturers. Carriers include glycerol (Grafton DBM, Osteotech, Inc., Eatontown, NJ), synthetic polymer (Dynagraft, GenSci OrthoBiologics, Inc., Irvine, CA), porcine gel (Osteofil, Regeneration Technologies, Inc., Alachua, FL), and carboxymethylcellulose (Allomatrix Injectable Putty, Wright Medical Technology, Inc., Arlington, TN). DBM is also available in a particulate powder form that has no carrier material present. This form is useful to admix to other materials such as iliac aspirate or autograft and platelet gels ( Fig. 4-4 ).

Bioactivity can be measured by in vivo assays (which measure de novo bone formation and alkaline phosphatase levels) and in vitro assays (which measure stimulation of human osteoblast culture).
Only a few tissue banks use an in vivo model to measure the bioactivity of the final DBM product with the carrier. A study by Han and colleagues evaluated the effects of moisture from water-based carriers and the storage temperatures on osteoinductivity of known DBM products. This was done to evaluate these materials with regard to shelf life in an operating room. In a dry state, without a carrier, DBM can preserve its osteoinductive activity when temperatures reached 65° C (149° F), but in the presence of moisture, (carrier substances) the activity decreases with incubation time. Nearly 90% of the DBM activity is lost when maintained for 5 weeks at 65° C (149° F).
While the preclinical data are impressive for DBM forming de novo bone in lesser animal models, the human clinical data are deficient with only isolated case reports and uncontrolled retrospective reviews. These level 3 and 4 studies suggest the potential therapeutic effects of DBM. The maxilla-craniofacial field published the bulk of the literature documenting successful reconstruction of complex deformities using DBM alone as a bone graft substitute. Reported success rates for mandibular and maxillary reconstructions are consistently more than 90%.
One of the first clinical series was published by Tiedeman and colleagues. They reported on an uncontrolled case series of 48 patients in whom DBM had been used in conjunction with BM for the treatment of skeletal injuries. Thirty-nine patients were available for follow-up, and 30 of them demonstrated healing. These results were encouraging; however, because there was no control group, the role of DBM in patients who demonstrated healing remains unknown. Unfortunately, most clinical series combined DBM with other adjuvants and, as noted earlier, the singular effectiveness of DBM alone is difficult to elucidate.
Two separate investigators evaluated the effectiveness of combining DBM with BM aspirate for the treatment of acute long bone fractures and nonunions. Wilkins and colleagues treated 66 patients with 69 “stiff” nonunions with a prospective protocol. The only therapeutic intervention was the percutaneous administration of a mixture of autologous BM and allograft DBM on an outpatient basis. Sixty-one of the percutaneous treatments (88%) resulted in union (level III evidence), however, there was no comparative control group other than historic controls with which to compare the effectiveness of this composite graft.
Lindsey studied the effectiveness of a composite graft consisting of DBM putty (Grafton DBM) and aspirated BM for treating long bone acute fractures. Patients were randomized to treatment with either the DBM putty composite or iliac crest autograft, with a minimum of 12 months of radiographic follow-up. Ninety percent of DBM patients achieved full bone formation compared to 75% of autograft patients ( P = 0.41). Additionally, all DBM patients were healed compared with 63% of autograft patients ( P = 0.07). Both of these studies suggest that DBM putty enriched with BM may be comparable to autograft for treating long bone fractures and nonunions.
However, in the case of acute fractures, the number of patients in each group was 10 or less, and although this was a randomized prospective trial (level I evidence), the numbers are too small to draw definitive conclusions regarding this composite graft option. Despite these limitations, both of these studies suggest that DBM putty enriched with BM may be comparable to autograft for treating long bone fractures and nonunions. This option offers the distinct advantages of decreased morbidity, reduced costs, and shorter hospital stay compared to AICBG.
There is only one level 1 randomized prospective study in humans available, evaluating the use of DBM as a solitary graft material. This study used DBM alone to treat a standardized critical-sized fibular defect, grafted using only DBM (positive control) compared to a similar defect with no graft applied negative (untreated) controls.
This was the first phase of a study done in conjunction with a prospective, randomized double-blind study in 24 patients undergoing high tibial osteotomy to evaluate the effectiveness of human recombinant osteogenic protein (OP-1) on a type I collagen carrier in a critically sized fibular defect (phase II). The results of phase I established the critically sized nature of the defect. In the defect group with no graft augmentation, no bony changes were observed while nonunion resulted in all of these defects. In the group grafted with DBM, formation of new bone was visible from 6 weeks onward with many defects undergoing complete healing. The results of the second phase showed no significant formation of new bone in the presence of collagen graft alone, while in the OP-1 group, all patients except one showed formation of new bone from 6 weeks onward. This study demonstrated that both DBM and OP-1 exhibited osteogenic activity in a validated critically sized human defect.
In a similar study, Hierholzer and colleagues attempted to evaluate the healing of humeral shaft nonunions using a consistent surgical protocol but compared the use of two different types of bone graft: autologous iliac crest bone graft and DBM in a consecutive retrospective cohort series. Forty-five patients were treated with AICBG and 33 grafted with DBM only. Union was noted clinically and radiographically in 100% of the 45 patients treated with autologous bone graft and 97% (32) of the 33 patients treated with DBM (not significant evidence). The overall functional outcome did not differ between the groups; however, 20 (44%) of the autologous bone graft recipients had donor site morbidity, including prolonged pain in the majority and a superficial infection requiring irrigation and débridement in 1 patient. These results demonstrated that consistent healing could be achieved with commercially available DBM alone (level III evidence).
Augmentation of spinal fusion appears to be the most frequent application of DBM. In the more challenging environment, such as posterolateral spine fusion, multiple formulations and combinations have been used. Significant differences exist between the various forms of DBM in their ability to generate spine fusion in animal models. This must be considered when analyzing the data from human studies.
In a side-by-side comparative study, a total of 120 patients underwent posterolateral spine fusion with pedicle screw fixation and bone grafting. Iliac crest autograft was implanted on one side of the spine and a Grafton DBM/autograft composite was implanted on the contralateral side in the same patient. The bone graft mass was fused in 42 cases (52%) on the Grafton DBM side and in 44 cases (54%) on the autograft side. The authors concluded from this level III study that DBM can extend a smaller quantity of autograft than is normally required to achieve a solid spinal arthrodesis. Consequently, a reduced amount of harvested autograft may be required, potentially diminishing the risk and severity of donor site complications.
Thus, the use of DBM as a bone graft extender appears to be a viable indication for its use.
In a larger 2-year prospective study, a randomized clinical trial compared the outcomes of Grafton DBM combined with local bone, with that of iliac crest bone graft (ICBG) in a single-level instrumented posterior lumbar fusion. Patients were randomly assigned (2 : 1) to receive Grafton DBM with local bone or autologous ICBG.
At 2-year follow-up, subjects who were randomized to Grafton DBM and local bone achieved an 86% overall fusion rate versus 92% (ICBG) ( P = 1.0, not significant evidence) and improvements in clinical outcomes that were comparable with those in the ICBG group. There was a statistically significant greater mean intraoperative blood loss in the ICBG group than in the Grafton group ( P < 0.0031).
A recent comprehensive meta-analysis reviewed the use of DBM for spinal fusion series. Articles were critically examined and compared according to study design, DBM type, outcomes, and results. The primary outcome of interest was fusion rate. Secondary outcomes included the Oswestry Disability Index, SF-36 survey, Odom’s criteria, visual analog scale (VAS), neurologic pain score, Japanese Orthopedic Association myelopathy score, Neck Disability and Ishihara Curvature indices, and pseudarthrosis and surgical failure rates. The majority of human clinical trials report high fusion rates when DBM is employed as a graft extender or a graft enhancer. Few prospective randomized controlled trials have been performed comparing DBM to autologous ICBG in spine fusion.
Although many animal and human studies demonstrate comparable efficacy of DBM when combined with autograft or compared to autograft alone, additional high level of evidence studies are required to clearly define the indications for its use in spine fusion surgeries and the appropriate patient population that will benefit from DBM.
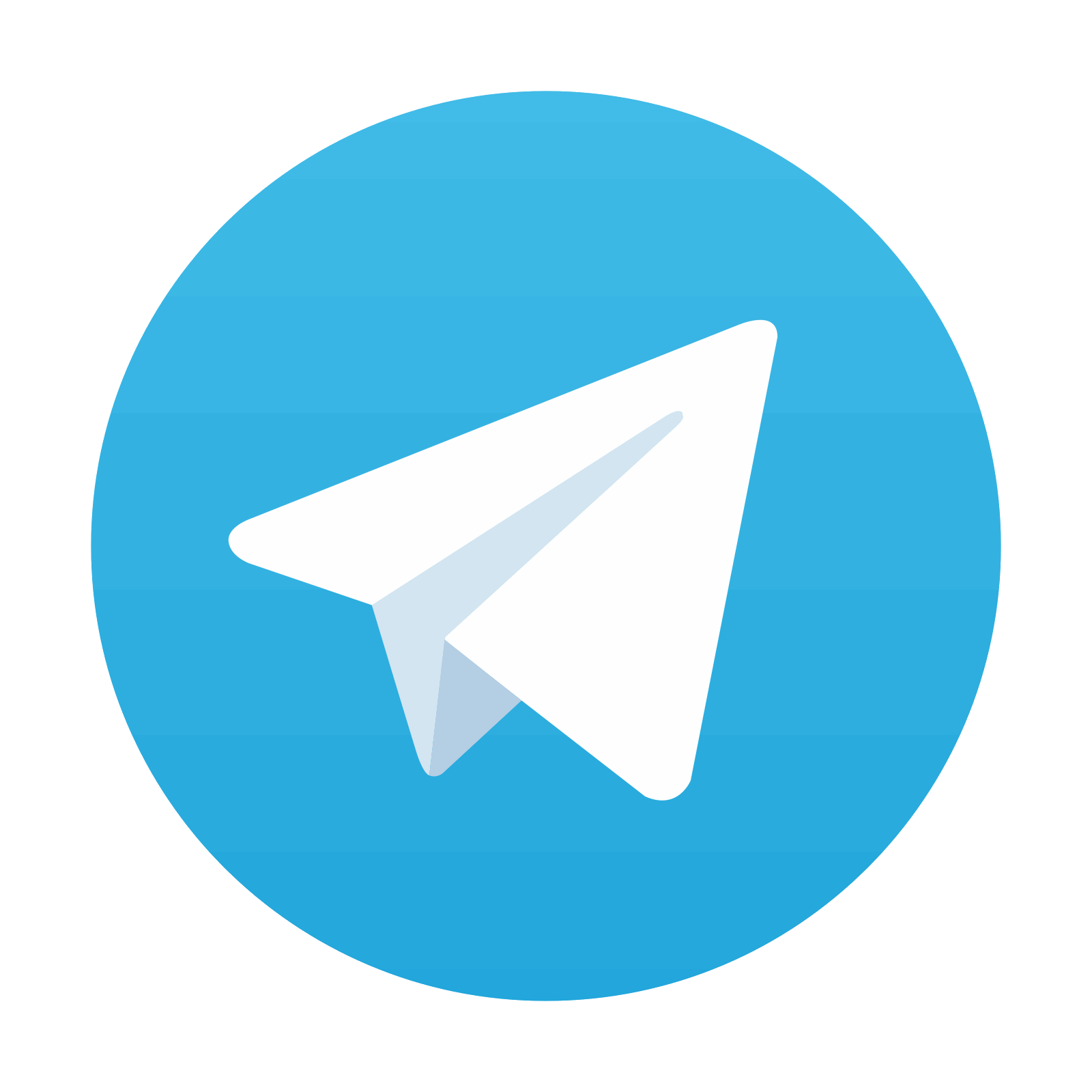
Stay updated, free articles. Join our Telegram channel

Full access? Get Clinical Tree
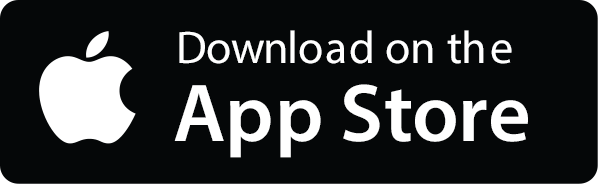
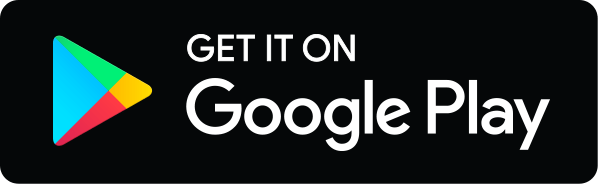