Basic Science of Spinal Fusion
Barrett S. Boody
Danielle S. Chun
Wellington K. Hsu
Introduction
Spinal fusion is a common adjunct of spinal surgical procedures to eliminate motion between vertebral segments. This process occurs from the result of bony deposition with subsequent remodeling and union between prepared vertebral surfaces. Although the pseudarthrosis rate has been historically reported at 5% to 40%, our improved understanding of host-related factors and further technologic innovations continue to decrease this incidence. Understanding the normal mechanisms of bone formation in spinal fusion, significant risk factors for pseudarthrosis, and surgical techniques and materials available for spinal fusion procedures is critical for successful outcomes.
Mechanism of Bone Healing
Successful fusions require robust quantities of viable osteogenic and progenitor cells, adequate osteoinductive growth factors, an osteoconductive matrix for bone growth, vascularity, a mechanically stable environment, and an appropriate inflammatory response. The surgical trauma to soft tissues during preparation of the fusion bed and placement of hardware should be minimized, as preserving local vascularity and healthy surrounding tissues is important to the initiation of the bony fusion process. The early initiation of the inflammatory response is critical to achieving successful fusion. The postoperative hematoma provides an influx of chemotactic and inductive cytokines, such as platelet-derived growth factor (PDGF), vascular endothelial growth factor (VEGF), and transforming growth factor-β (TGFβ). Paracrine signals, including cytokines, prostaglandins, and kinins, act as local chemotactic signals for subsequent stages of fusion and stimulate angiogenesis to vascularize the nascent fusion bed. Local debridement of necrotic tissues by neutrophils and macrophages prepares the area for the development of a fusion mass.
Preparation and decortication of the fusion bed provides a supply of stem cells from the marrow as well as exposure of the robust cancellous intraosseous vascular supply for the anticipated fusion. The three main areas commonly used for fusion are interbody, posterolateral (intertransverse process region), and interlaminar–facet joint region. The transverse processes are a major source of vascularity for posterolateral fusion. This area is decorticated to expose the well-vascularized cancellous bone. The pars interarticularis should be avoided, as the region provides structural support and is largely devoid of sufficient cancellous bone. Decortication of the posterolateral spinal elements, notably the transverse processes, can be performed with hand instruments (rongeurs and osteotomes) or with an oscillating burr. Increasing the surface area facilitates the local release of osteogenic cells and osteoinductive signals and provides a larger foundation for the future fusion mass.
Following surgical preparation of the fusion bed, bone graft is placed. A multitude of options exist for facilitating bony union. While there is no consensus on what the optimal graft is, the historical gold standard remains iliac crest autograft.
While autologous bone is considered to contain a robust amount of osteogenic cells, the viability of the graft is limited by the recipient site’s vascularity. Commonly, only a small fraction of the transplanted cells survive. Prior to vascularization of the fusion mass, the cells rely on local diffusion for nutrition after implantation, with surviving cells likely within the 1- to 2-mm outer periphery. Necrotic cells must then be cleared in preparation for osteogenesis, requiring an influx of inflammatory cells and further compromising the already precarious blood supply to the transplanted graft cells. Accordingly, excessive administration of osteogenic cells may delay or inhibit fusion due to excessive bioburden of necrotic graft. However, the optimum amount of bone graft to promote fusion is not well known and is likely contingent on the site and size of anticipated fusion mass as well as significant personal experience.
Boden et al. characterized the process of spinal fusion as preceding through inflammatory, reparative,
and remodeling stages. The inflammation phase begins with the postoperative hematoma surrounding the graft material. The subsequent influx of inflammatory cells and neovascularization facilitates initial membranous ossification at the transverse processes. The subsequent reparative phase begins with remodeling of the early ossification at the transverse processes with resorption of necrotic bone graft. Maturation of the fusion mass begins at the edges of the transverse processes with ossification subsequently seen later at the central zone. Endochondral ossification bridges the fusion “outside in” with a cartilage template formed by roughly 3 to 4 weeks. The final remodeling stage begins with further resorption of the bone graft and replacement of the initial bridging fusion mass with trabecular bone. The cancellous to cortical bone ratios of the fusion mass decrease throughout the remodeling process as the fusion mass more closely resembles cortical bone.
and remodeling stages. The inflammation phase begins with the postoperative hematoma surrounding the graft material. The subsequent influx of inflammatory cells and neovascularization facilitates initial membranous ossification at the transverse processes. The subsequent reparative phase begins with remodeling of the early ossification at the transverse processes with resorption of necrotic bone graft. Maturation of the fusion mass begins at the edges of the transverse processes with ossification subsequently seen later at the central zone. Endochondral ossification bridges the fusion “outside in” with a cartilage template formed by roughly 3 to 4 weeks. The final remodeling stage begins with further resorption of the bone graft and replacement of the initial bridging fusion mass with trabecular bone. The cancellous to cortical bone ratios of the fusion mass decrease throughout the remodeling process as the fusion mass more closely resembles cortical bone.
Variable gene expression at the fusion site tightly regulates the progression of spinal fusion. Three bone morphogenetic proteins (BMPs-2, -4, and -6) are commonly and reproducibly seen throughout the fusion process. The presence of BMP-6 mRNA peaks both early (day 2) and late (week 5), BMP-4 mRNA peaks at week 1, and BMP-2 mRNA peaks at week 3, with each unique peak suggesting unique and vital roles in bony fusion. Furthermore, the BMP expression throughout the fusion bed displays significant spatial heterogeneity, with the periphery displaying earlier BMP gene expression than the center of the fusion mass. The spatial variability in BMP gene expression in spinal fusions explains the clinical lag in healing seen at the center of the fusion mass and identifies an opportunity for osteoinductive proteins to circumvent a vulnerable timepoint for successful graft fusion. Morone et al. found the addition of BMP-2 to autograft in rabbit posterolateral spinal fusion models accelerated the gene expression of BMP-6, type I collagen, and osteocalcin along with other markers of early bone formation within the central fusion mass, eliminating the lag effect seen in the control group.
Bone Grafting Core Concepts
The optimal bone graft material displays the following properties:
Osteoconductivity
Osteoinductivity
Osteogenicity
While the term “bone graft” commonly refers to autograft or allograft (Table 37.1), a variety of graft materials are available to serve as bone graft extenders, enhancers, or substitutes. An extender is typically only efficacious when added to autograft or other type of stand-alone graft. These products can increase the volume of the graft past a critical threshold in which bone healing occurs which correlates positively with fusion rates. An enhancer augments the graft’s potency or efficacy without necessarily increasing the graft volume. A commonly used example of a bone graft enhancer is BMP, a growth factor with strong osteoinductive capabilities that is frequently added to allograft or autograft to improve fusion rates. Finally, a substitute can independently facilitate all of the critical elements of bone repair and can be used in place of autograft. Bone grafts may be utilized differently depending on the fusion site. For example, allograft functions as a substitute in the anterior cervical spine but only as an extender in the posterior lumbar spine.
TABLE 37.1 BONE GRAFT TYPES AND PREPARATIONS COMMONLY USED IN SPINAL SURGERY | ||||||||||||||||
---|---|---|---|---|---|---|---|---|---|---|---|---|---|---|---|---|
|
The osteogenic potential of the graft is directly related to the amount of viable transplanted osteoblasts or precursor progenitor cells that are capable of osteoblastic differentiation. Most commonly, the transplanted surface osteoblasts on cancellous and cortical bone are responsible for producing new bone at the fusion site. Autograft and bone marrow aspirates (BMAs) provide these constituents, unlike other grafts such as structural allograft and ceramics.
Bone graft options may also display osteoinductive properties, defined as the ability to promote differentiation, recruitment, and migration of adjacent progenitor cells provided by the hematoma, local inflammation, adjacent soft tissues, or prepared fusion bed into an osteogenic cell line. The most studied, and currently the most potent osteoinductive signals are the BMPs, a subset of the TGFβ family of growth factors (Fig. 37.1). Although a variety of growth factors are necessary for successful fusion (PDGF, TGFβ, VEGF, etc.), BMPs
used in isolation have uniquely demonstrated the ability to facilitate ectopic bone formation.
used in isolation have uniquely demonstrated the ability to facilitate ectopic bone formation.
Osteoconduction refers to the physical properties of the graft that facilitates the attachment of osteogenic cells, migration of cells along the structure, and ingrowth of vascularity throughout the scaffold at the proposed fusion site. Its efficacy is largely dictated by pore size, surface texture, adhesive properties, rate of resorption, and the ability to bind osteoinductive proteins. Recent applications and studies have shown clinical success using osteoconductive materials to deliver osteoinductive proteins. While autograft and allograft bones display sufficient osteoconductive capacity, a variety of commercially available products such as collagen, ceramics, coral byproducts, and other polymers also provide these characteristics. Ceramic materials are commonly used in orthopedic procedures and are comprised of natural coral (calcium carbonate), coralline hydroxyapatite, or composites (calcium triphosphate). Because the chief limitation of these products is a lack of osteoinductivity, they are primarily used as extenders.
Risk Factors for Nonunion
Host risk factors for nonunion and/or delayed union:
Tobacco use (primarily smoking tobacco)
Dosage and potency of nonsteroidal anti-inflammatory drug (NSAID) use
Osteoporosis
Multilevel and revision fusion procedures
Immunosuppression
Smoking
Multiple studies have linked cigarette smoke to delayed union and pseudarthrosis in spinal fusion procedures with at least a 10% to 12% decrease in lumbar fusion rates. Failure to quit smoking postoperatively also further decreases bone healing potential. However, several investigators noted successful outcomes with spinal fusion in smokers undergoing one-level anterior cervical discectomy and fusion (ACDF) with allograft and plate fixation, posterior cervical fusions with instrumentation, cervical autograft strut grafting for multilevel cervical fusions, and lumbar interbody fusions with BMP. Additionally, it is still unclear what the residual effects of cigarette smoke are after successful cessation. While the effects of cigarette smoking and cessation continue to be elucidated for spinal fusion, it is reasonable to conclude that smoking is detrimental to spinal fusion and can promote pseudarthrosis in at-risk hosts and fusion locations.
Osteoporosis
Osteoporosis demonstrates a significant challenge in successful spinal fusion. The reduction in bone mass and altered microarchitecture of the cancellous and cortical bone in the osteoporotic spine potentially lead to screw loosening and impaired fusion rates due to decreased mechanical stability. Additionally, the reduced osteogenic potential of mesenchymal stem cells in osteoporotic patients further impairs successful spinal fusion. Evaluating the effects of bone mineral density (BMD) on posterolateral interbody fusions with supplemental pedicle screws fixation, Okuyama demonstrated a significant correlation between higher rates of screw loosening and nonunion with lower BMD levels.
There are a number of pharmacologic options for the treatment for osteoporosis. The use of bisphosphonate medications during spinal fusion has demonstrated mixed results in animal models. Several preclinical studies have demonstrated lower rates of successful fusion, decreased stiffness of fusion mass, and less histologic evidence of bony union with alendronate treatment. However, other similar preclinical trials have suggested no detrimental impact of bisphosphonates on the biomechanical strength of fusion masses. Human trials on the impact of bisphosphonates on spinal fusion are sparse,
however. Nagahama et al. demonstrated an increased fusion rate after posterior lumbar interbody fusions (PLIFs) in osteoporotic patients treated with alendronate (95% vs. 65% in controls), suggesting improved mechanical stability overcame any adverse biologic effects on bone fusion.
however. Nagahama et al. demonstrated an increased fusion rate after posterior lumbar interbody fusions (PLIFs) in osteoporotic patients treated with alendronate (95% vs. 65% in controls), suggesting improved mechanical stability overcame any adverse biologic effects on bone fusion.
Zoledronic acid (ZOL) is a nitrogen-containing bisphosphonate that displays a higher affinity for hydroxyapatite compared to other members of the nitrogen-containing bisphosphonates. In a clinical study, ZOL led to increased fusion rates (75% vs. 56%), improved disability and pain scores, lower incidence of osteoporotic vertebral compression fractures, screw loosening and subsidence, as compared to controls.
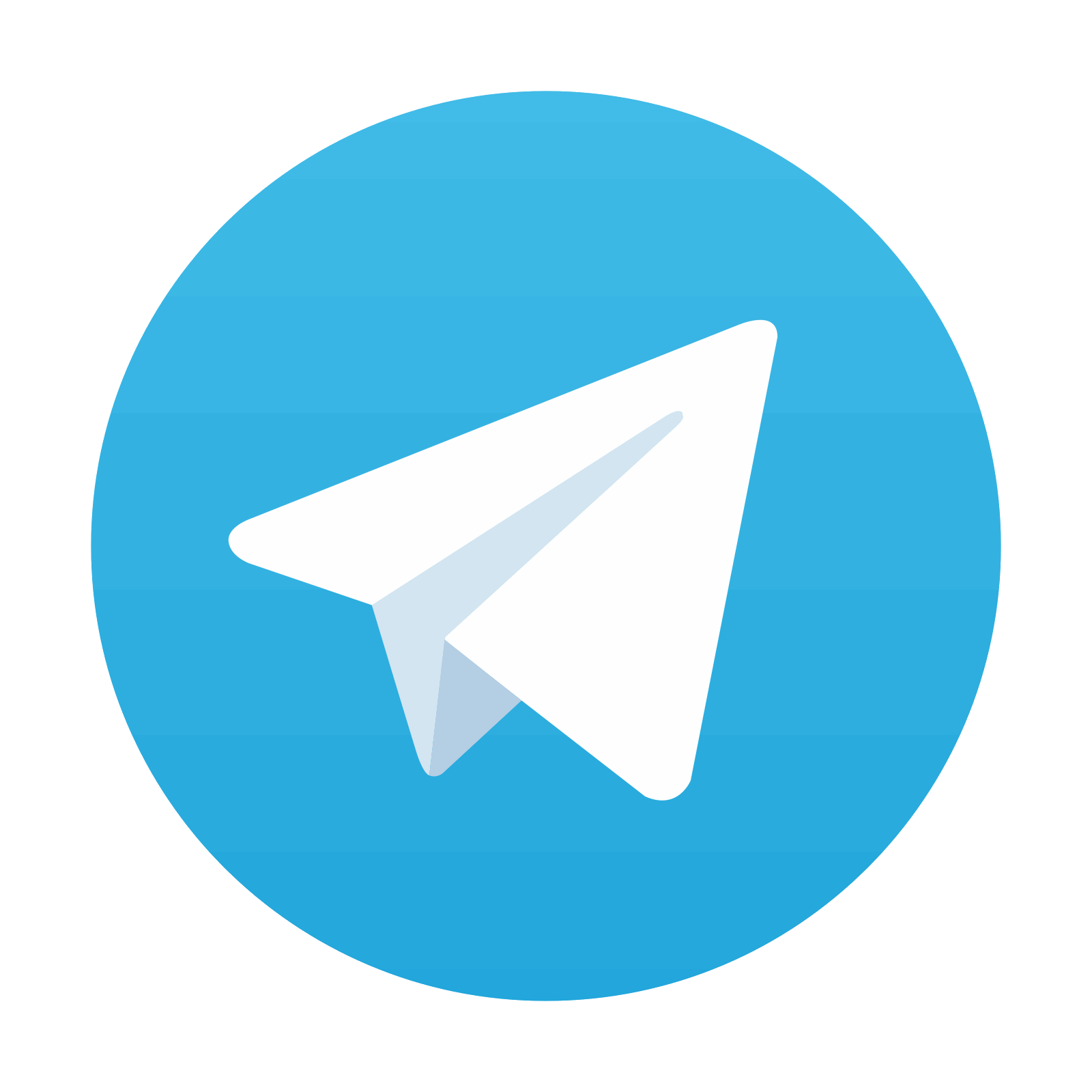
Stay updated, free articles. Join our Telegram channel

Full access? Get Clinical Tree
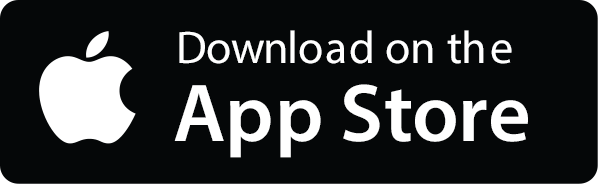
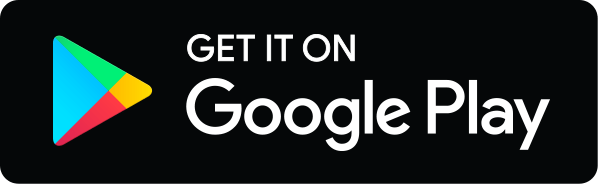