PAIN REHABILITATION CAN BE DEFINED AS THE process of helping an individual achieve the highest level of function, independence, and quality of life possible through the management of a painful condition and its impact on daily activities. Pain is a complex phenomenon that requires a functional approach taking into account physiological, psychological, and contextual domains. Multidisciplinary biopsychosocial rehabilitation is generally the model used for chronic pain with a superior effect on pain reduction and quality of life compared to disciplinary approaches or to surgery.1 Considering its multifaceted aspect, it is not surprising that rehabilitation is often the most important approach in the treatment of pain.
Understanding the neurophysiology of pain is essential not only for the clinician, but also for the patient. In a recent study, the authors found that patients with higher knowledge in pain neurophysiology have less fear avoidance and lower perceived disability due to pain.2 It is then essential for any clinician working with patients suffering from chronic pain to have a good background of pain neurophysiology to transfer this empowering knowledge to the patient.
In this chapter, we will introduce the basic anatomy and physiology of pain with an emphasis on endogenous excitatory and inhibitory mechanisms. We will discuss the mechanisms implicated in the development, persistency, and treatment of pain with an emphasis on the role of rehabilitation. The main goal is to introduce pain neurophysiology in functional terms so the clinician can appropriate and apply the concepts in a clinical environment.
The ideal goal in pain rehabilitation is to identify the cause, utilize an appropriate treatment, decrease pain perception, and improve function. Generally, the efficacy of treatment is limited by the presence or absence of an accurate diagnosis. In chronic pain, however, a clear diagnosis is rarely identified; the etiology of chronic pain is often complex and multifactorial. The combination of pharmacological and nonpharmacological approaches is used to alleviate suffering and improve function. Rehabilitation has always played a major role in the control of chronic pain.
Exercise is known to be one of the most important aspects of pain rehabilitation.3 However, fear avoidance belief leads to kinesiophobia, which is a major factor of poor outcomes in chronic pain.4 One way to reduce the fear avoidance belief is to educate patients about the neurophysiology of pain.2 This knowledge will help make it clear that several nonpharmacological approaches have solid physiological grounds and are frequently essential in the treatment of pain.
Patient collaboration is a major element in the treatment of chronic pain. Their involvement is essential to get the best results. Understanding the neurophysiological mechanisms of pain will guide the clinician to choose the best treatment for a specific condition, but will also help educate the patient to become a collaborator in the treatment team.
Pain is a dynamic phenomenon. The association between nociceptive activity and pain perception depends on several intrinsic and extrinsic influences. For the same nociceptive stimulus, pain perception and related brain activity will greatly differ between subjects.
The importance of intrinsic factors in pain is supported by genetic predispositions to be less or more sensitive to pain.5 External factors such as a stressful or anxiogenic event will influence our perception of pain and will even change how our brain reacts to painful events.6 External and intrinsic factors are interacting. Epigenetics, lasting changes in gene expression without alteration of the DNA sequence, are triggered by external factors and affect pain perception.7 Nerve injuries or even psychological factors could change the central nervous system by affecting DNA methylation and produce a “genomic” memory of pain in the adult cortex.8
Fortunately, positive effects of extrinsic factors can also happen. The practice of exercise, meditation, or yoga could produce some brain activity changes that will help reduce chronic pain conditions.6 Altogether, this information is underlining the major role of rehabilitation in the treatment of chronic pain.
The evolution of our understanding and management of pain is driven by theories. It is then interesting to have a brief overview of the evolution of our understanding of pain mechanisms.
The specificity theory was first introduced by Descartes during the seventeenth century9 and was refined with modern physiology by Müller and Frey at the end of the nineteenth century.10,11 They proposed that the somatosensory system could be divided according to specific receptors for tactile, hot, cold, and pain receptors. Specificity is our theoretical framework to explain how nociceptive afferences from the periphery connect to specific pathways projecting to cortical structures that are related to sensory and affective components of pain.12 Even if several studies support that these pathways and higher center structures are definitely playing a role in pain perception, their anatomic identification is not sufficient to explain the complexity of pain.
The pattern theory, introduced by Goldscheider,13 suggested that in addition to the type of input (fibers, pathways, or the different anatomic structures), the pattern of impulses in the nervous system modulates pain perception. Based on this theory, it is easier to understand that a thermal stimulus can go from a warm perception to burning hot if the stimulation persists at the same temperature (temporal summation) or is presented on a larger surface (spatial summation).
Changes in the activation patterns could help understand complex phenomena such as allodynia, pain from a nonpainful stimulus, or spontaneous pain in conditions where no apparent lesions are detectable. We understand that even small changes in the neuronal activity of spinal or supraspinal structures will be sufficient to produce what is now known as central sensitization. Central sensitization can be described as the plasticity of the central nervous system that will produce a reduction of the threshold to produce a painful sensation to the point that even a non-nociceptive stimulus will be perceived as painful (allodynia) or a nociceptive stimulus will be perceived as more painful than usual (hyperalgesia), as well as a receptive field expansion that will enable the noninjured tissue to produce pain (secondary hyperalgesia).14
In 1965 the gate control theory by Melzack and Wall15 came with another important part of the complex puzzle of pain: the fact that endogenous pain modulatory mechanisms could enhance or reduce pain perception. For instance, the gate control theory proposed that the stimulation of nonpainful Aβ afferences could produce a localized analgesia by blocking the nociceptive afferences directly at their entry in the spinal cord. Moreover, even if the specific mechanisms were not explained in the gate control theory, Melzack and Wall already proposed that descending mechanisms from higher centers would influence this modulatory mechanism.
A few years after the gate control theory was proposed, Reynolds demonstrated that stimulation of the periaqueductal gray (PAG) in the brainstem produced a strong inhibition.16 The role of the rostroventral medulla in the modulation of pain has since been well documented.17 Regions such as the PAG and the nucleus raphe magnus (NRM) have been identified as important serotoninergic and noradrenergic descending inhibitory pathways. These inhibitory pathways then recruit enkephalinergic interneurons in the spinal cord to produce the analgesic response.
The diffuse noxious inhibitory controls (DNIC) model was proposed in the 1970s.18,19 This model is based on the observation that a localized nociceptive stimulation can produce a diffuse analgesic effect over the rest of the body, an analgesic approach known as counter-irritation. In the DNIC model, Le Bars and his colleagues18 proposed that a nociceptive stimulus will send input to superior centers, but will also send afferences to the PAG and NRM of the brainstem, recruiting diffuse descending inhibitory output at all levels of the spinal cord.
Together, the gate control and DNIC have played a very important role in supporting the idea that pain perception is not only the endpoint of nociceptive activation but is modulated by several endogenous mechanisms. Deficits of these mechanisms are probably responsible for several complex chronic pain conditions.20
Another very interesting view of pain has been proposed by Bud Craig.21 Rather than seeing pain as part of the exteroceptive sense of touch, he suggests that we have neuroanatomic and neurophysiological demonstrations that it is, in fact, a homeostatic signal. The human experience of pain is then both a distinct sensation and a motivation at the same time. This model makes sense when we think that pain is described as a sensory, affective, and cognitive experience. The International Association for the Study of Pain (IASP) definition of pain as “an actual or potential lesion” fits the homeostatic and behavioral drives concept. Moreover, lesions of the somatosensory cortex often do not induce pain, while thalamic stimulation has been shown to produce analgesia.21 The earliest brain activity following a nociceptive stimulus is in the posterior insula and midcingulate cortex,22 two regions that are playing a role in the affective reactions and in homeostasis.
Most of the rehabilitation approaches are derived from the different theories that are briefly described in this section. For example, massage, transcutaneous electrical nerve stimulation (TENS), psychological reframing, and mirror therapy are based on applications of the previously noted theories.
For a brief review of the neuroanatomy and neurophysiology of pain, we will follow the nociceptive signal from the periphery to the cortex. It is also important to recognize the role of descending signal from the higher centers to the brainstem and the periphery that will modulate the nociceptive signal at all the levels of the central nervous system, constructing pain perception.
The term “nociception” comes from Sherrington’s observations regarding stimuli that are likely to affect the integrity of the body.23 It indicates potentially painful or algesic nerve information before it comes to consciousness or higher brain centers. Frequently a nociceptive stimulus will produce pain. However, several conditions can change this perception, depending on the salience or significance of the information.24 In a neutral condition, pain is normally very salient. It’s a protective mechanism. However, in an emergency or during an important distraction, pain salience may shift to a second order and will be felt as lower or even absent.
A nociceptive stimulation will recruit peripheral nociceptors that conduct the nociceptive signal in the primary somatosensory neuron to the dorsal horn of the spinal cord (Fig. 34–1). In the dorsal horn, the primary afferent neuron will make a synaptic contact with secondary or projection neurons. Secondary neurons from the spinothalamic (lateral) and spinoreticular (medial) tracts will immediately cross in the spinal cord and send afferent projections to higher centers. A large proportion of afferents will make a second synapse in the lateral and medial nuclei of the thalamus, which subsequently make synaptic contact with tertiary neurons. It is important to emphasize that the secondary neurons may also synapse with neurons in different nuclei of the brainstem, including the PAG and the NRM, areas involved in descending endogenous pain modulations. Tertiary neurons from the thalamus send afferents to the primary and secondary somatosensory cortices (S1, S2). The S1 and S2 are involved in the sensory quality of pain, which includes location, duration, and intensity. Tertiary neurons also project to limbic structures, including the anterior cingulate cortex (CC) and the insula, which are involved in the affective or emotional component of pain.
Figure 34–1
Pain pathways. From the periphery to the cortex, we can follow the lateral spinothalamic (broken line) and the spinoreticular (full line) from the periphery to the cortex. The lateral spinothalamic tract project to the lateral thalamus nuclei and the somatosensory cortex. The spinoreticular tract is projecting to the medial thalamus and different cortical structures associated to the affective component of pain including, but not restricted to, the insula and the cingulate cortex. These different pain pathways are responsible for the complex pain-related perception.

Whenever one of the three levels of nociceptive neurons is making a synaptic contact, there is the integration of information that undergoes excitatory and inhibitory influences. These areas of integration are the targets of most analgesics. The nociceptive information that reaches the higher centers has undergone many excitatory and inhibitory influences at all levels of the central nervous system. The last step, the perception of pain, is the translation from a noxious stimulus to pain perception. However, pain perception can be present without peripheral nociceptive inputs and will be colored by emotions and the sum of the subject’s experiences.
To explain the physiological mechanisms of pain, we will briefly see the steps by which nociceptive information must pass before reaching consciousness. This neurophysiological knowledge is essential to understand the phenomenon of pain and its modulation.
The afferent fibers originating in the periphery fall into three groups, namely Aβ, Aδ, and C fibers.
The Aβ fibers are large myelinated fibers that conduct at high speed (35 to 75 m/s) and usually transmit non-nociceptive signals. They do, however, also participate in pain modulation by recruiting inhibitory interneurons in the substantia gelatinosa (SG) of the dorsal horn in the spinal cord. This mechanism is one of the fundamental components of the gate control theory, whereby an innocuous stimulus will reduce the nociceptive input from the same region.15 Besides playing a dynamic inhibitory role when recruited, the Aβ fibers also seem to play a tonic inhibitory role on the nociceptive input. Blocking the input from these large fibers will result in an increased response to nociceptive stimuli.25
Two other classes of fibers, the myelinated Aδ and the thin unmyelinated C fibers, mainly transmit nociceptive messages. The Aδ fibers are myelinated and relatively large, conducting the signal relatively rapidly (5 to 30 m/s) from the periphery to the spinal cord. Because of the rapid conduction velocity, the Aδ fibers are responsible for a rapid pinprick-like, sharp, and transient sensation. They represent the majority of the myelinated fibers. Two types of Aδ fibers exist depending on the specificity of their responses to different stimulation26: (1) the mechanonociceptors respond preferentially to intense and potentially harmful mechanical stimulation, and (2) the polymodal Aδ fibers respond to mechanical, thermal, and chemical stimulation.
In contrast, the C fibers that have a slow conducting velocity (0.5 to 2 m/s) will mediate a second or dull, aching pain. They represent three-quarters of the sensory afferent input and are mostly recruited by nociceptive stimulation. Because of their slow conduction velocity, they are responsible for the second pain: a dull, diffuse, and late sensation. However, they are also involved in non-nociceptive somatosensory information such as in the sensation of itch (pruritus).27 Paradoxically, the C fibers are also involved in the perception of pleasant touch, as documented in a patient with a rare disease linked to a deafferentation of the myelinated sensory fibers.28
As discussed in the section on endogenous pain modulation, the inhibition of pain through the stimulation of non-nociceptive afferents primarily is described by the gate control theory,15 in which the selective recruitment of non-nociceptive afferents (Aß) inhibits nociceptive afferents (A∂ and C) by the recruitment of inhibitory interneurons in the SG of the posterior horns of the spinal cord. Clinically, this theory proposes that nonpainful peripheral stimulation on a specific region produces an analgesic effect in the same place. An example of this stimulation is the analgesia experienced when one rubs a painful region after injury. Several therapeutic approaches also fit this description, the most common being light massage. Many devices have been developed to produce analgesia based on this mechanism, either by vibration or by TENS. As we will see in the next paragraph, the localized analgesic effect takes place in the spinal cord.
The first major distinction between nociceptive and non-nociceptive afferent fibers is that the latter ascend ipsilaterally (on the same side) up to the brainstem before making synaptic contact with the second neuron and finally crossing to the opposite side before projecting to higher centers. For nociceptive fibers, the signal is transported to the dorsal horns of the spinal cord (or the brainstem for trigeminal afferent impulses) to make first synaptic contact with the secondary neurons (or projection neurons). The secondary neurons cross the spinal cord immediately under the central canal to form the spinothalamic contralateral projection tract.
The dorsal horn remains the preferred site for significant synaptic convergence. In fact, the same fiber from the dorsal horn of the spinal cord can receive cutaneous, muscular, and visceral afferent impulses.29 The convergence of afferent impulses originating from different systems allows us to better understand the interaction that can exist between systems that seem independent at first (see Fig. 34–2). Therefore, muscular pain could be exacerbated by a new visceral pain, and vice versa. This information is especially important for rehabilitation since an intervention aimed at reducing somatic pain may have an effect on visceral pain. Therefore, it is not surprising that techniques such as TENS could have positive effects on visceral pain such as dysmenorrhea30 or angina31 even if the current is superficial and will not affect directly the viscera.
Figure 34–2
The convergence-projection hypothesis of referred pain. According to this hypothesis, visceral afferent nociceptors converge on the same pain-projection neurons as the afferents from the somatic structures in which the pain is perceived. The brain has no way of knowing the actual source of input and mistakenly “projects” the sensation to the somatic structure. (Rathmell JP, Fields HL. Pain: Pathophysiology and Management. In: Kasper D, Fauci A, Hauser S, Longo D, Jameson J, Loscalzo J, eds. Harrison’s Principles of Internal Medicine, 19e New York, NY: McGraw-Hill; 2014.)

The dorsal horns contain an important network of synaptic convergences, bringing together the collateral fibers and interneurons. Thus, the transition through the sensory spine is an important step during which nociceptive information will be modulated. Its complex network of neurons, which includes endings of primary nociceptive neurons, secondary neurons, interneurons, and neurons of descending tracts, contains a multitude of neurotransmitters and a sizable mosaic of receptors that will modulate the nociceptive afferent impulses before they are forwarded to the higher centers.
The transmission of a nociceptive impulse is not summarized solely as the passage of nociceptive information between the first afferent neuron and the second projection neuron in the spinal cord. Excitatory and inhibitory interneurons actively participate in the modulation of nociceptive responses. Glial cells also play a dominant role in nociceptive response.32 The action of these excitatory and inhibitory neurons in the spinal cord could lead to central sensitization, or hyperalgesia.
Hyperalgesia is defined as an exaggerated response to normally painful stimulation. In the 1950s, Hardy and colleagues proposed that two kinds of hyperalgesias could affect the skin: primary hyperalgesia, occurring directly at the injury site, and secondary hyperalgesia, with its origins in the central nervous system (CNS).33
Primary hyperalgesia can be explained by the release of different inflammatory factors in the periphery, which leads to the recruitment of nociceptors near the site of the injury (potassium, prostaglandins, bradykinin, histamine, substance P, and serotonin), which has the effect of recruiting nearby nociceptors and producing sensitization. The injury site as well as the neighboring tissues will thus have lower pain thresholds.
Secondary hyperalgesia, on the other hand, can be explained by a central phenomenon that is known by the general term “central sensitization.”14 Repeated recruitment of C fibers after an injury can cause a series of events at the spinal level, which could have the effect of sensitizing the projection neurons in the dorsal horns of the spinal cord. High-frequency recruitment of C fibers will produce an increase in the action potential of the spinal neurons called wind-up.34
Wind-up is a relatively short-lived phenomenon, but the repeated recruitment of C fibers can also lead to spinal sensitization, which may extend over several hours or even several days.35
The identification of the source of hyperalgesia is mandatory since a patient suffering from primary hyperalgesia (nociception and inflammation) may have a good response to an anti-inflammatory medication, while if the patient is suffering from secondary hyperalgesia (central sensitization) she or he may require a treatment that will have a central effect on the neuronal hyperactivity such as anticonvulsants and sensory rehabilitation.
Before projecting their axons toward the higher centers, secondary neurons of the spinothalamic and spinoreticular tracts project toward the thalamic nuclei that can be divided into two groups: nuclei of the ventrobasal complex (VPL, VPM) and those of the centromedian (CM) or intralaminar complex. The nuclei of the ventrobasal complex mainly receive their afferents from the spinothalamic tract and project in turn toward the primary (S1) and secondary (S2) somatosensory cortex. The sensory-discriminative component of pain, that is, information about the location and identification of the painful stimulus (its nature and intensity), is attributed to these somatosensory projections. The CM nuclei mainly receive their afferents from the spinoreticular tract and project in turn toward the various structures of the limbic system. In this area, impulses originate from deeper laminae, through the spinothalamic tract and the paramedial tract (spinoreticulothalamic tracts and collaterals of the deep laminae of the spinothalamic tract). Fibers of this region emit signals in several areas of the ipsilateral cortex, particularly in the frontal lobe and the limbic system.36 These last two targets are also responsible for the motivational-affective component of pain, a component associated with an unpleasant sensation and the desire to escape from the suffering.
This simplified division allows us to understand how, relatively early in the CNS, the various pain pathways project into regions that are specialized, serving either the sensory-discriminative component (spinothalamic tract) or the motivational-affective component (spinoreticular tract).
The visceral system is a very sophisticated sensory system implicating the concomitant activity of two extrinsic innervations, vagal and spinal, as well as numerous intrinsic neurons.37 For example, the intestine has a neuronal system that operates independently but also in relation with the rest of the CNS, known as the brain–gut axis. Several visceral pain syndromes, such as irritable bowel syndrome, present no clear lesion or dysregulation of the painful organ. The brain–gut axis seems to play an important role in these syndromes and may help to better understand the interaction between external events such as a stressful situation and an effect on the symptoms.38 Emerging data also stress the importance of the microbiome and the significance of a microbiome–brain–gut axis in some pathological conditions, including pain.39 These results suggest that alteration in the gut microbial composition is associated with marked changes in behaviors such as mood, pain, and cognition that are related to a bidirectional communication between the brain and the gut microbiota.40 Understanding these interactions may lead to treatments acting on the microbiota that will affect brain functions.
As for somatic pain, chronic visceral pain is related to both peripheral and central sensitization. Excitatory and inhibitory descending pathways are also implicated in the visceral system, suggesting an important central influence of visceral sensitivity. Finally, the autonomic nervous system’s influence on visceral sensitivity may help explain the role of emotions on the modulation of visceral pain. Based on these observations, some chronic visceral pain presents the characteristics of neuropathic pain.37
Interestingly, we all have experienced what we call a “gut feeling,” for example, a situation that feels uncomfortable without being able to clearly identify why. James, at the end of the nineteenth century,41 already proposed that body responses are fundamental to perceive emotions. More recent research supports the importance of our body’s interpretation of external stimuli, which is encoded in the insula and plays an important role in general emotional states, including our analysis of a pain state.42,43 This close interaction between visceral afferences and the insular cortex may help understand why visceral pain has such important emotional effects.
The role of the cortex in pain perception has been demonstrated relatively recently.44,45 Because an animal cannot tell us its perception of pain, one must refer to its nociceptive behaviors, suspecting that these behaviors are pain responses.
Imaging studies have reported increased pain-associated activity in a number of brain sites, including sensory (primary and secondary somatosensory cortices [SI, SII]), affective (anterior and medial cingulate cortices [ACC/MCC], insula, prefrontal cortex [PFC]), cognitive (ACC/MCC, PFC, SII), and motor (supplementary motor area [SMA], cerebellum).46,47 However, it has been proposed that there is no specific pain matrix since activities in these regions can also be recorded by different stimulation modalities that are not painful and could then be more related to the salience of the stimuli rather than specific to pain.48 Interestingly, Garcia-Larrea and Peyron46 proposed that there are at least three pain matrices which are responsible for our complex pain experiences: (1) the cortical nociceptive matrix, (2) the perceptual matrix, and (3) the pain memory matrix. These are related to specific brain regions related to different aspects of pain perception.
The cortical nociceptive matrix projects from the posterior thalamus nuclei to the posterior insula, medial parietal operculum, and mid–cingulate cortex. This first-order matrix is the earliest response to noxious stimuli.
The perceptual matrix is composed of several cortical regions, including the mid and anterior insula, anterior cingulate, prefrontal cortex, and the posterior parietal area. This perceptual matrix is different from the nociceptive matrix by the fact that it doesn’t receive direct nociceptive inputs and it can be activated in contexts not involving pain. It is a context-dependent matrix.
The pain memory matrix is composed of several high-order cortical structures such as the perigenual cingulate, the orbitofrontal cortex, the temporal lobe, and the anterolateral prefrontal cortex. Important changes in pain perception can occur without any nociceptive stimuli and without changing the activities in the pain pathways from the thalamus to the somatosensory cortex, for instance. A good example is the empathetic observation of someone in a painful situation that will make one grimace and feel as if they themselves were experiencing their pain.49 Manipulating the unpleasantness of a painful stimulus by giving it a different meaning, being the less intense or more pleasant versus being the most intense or more unpleasant in a series of stimuli, modulates the perceived intensity by changing the activity in higher-order structures of this matrix.50
It is artificial to present different brain structures as being responsible for a specific pain component since each of these structures is influencing how the other structures of the pain matrix will code the message. For the sake of understanding the neurophysiology and the pathophysiology of pain, it is still interesting to try to understand the neuroanatomic organization of the higher structures that are playing different roles in the experience of pain, keeping in mind that it is not a static but rather a dynamic system.
Since the first studies of cerebral imagery of the regions that play a role in pain using positron emission tomography (PET),51 several subsequent studies have confirmed the participation of the four principal cerebral centers (Fig. 34–3): the primary somatosensory cortex (S1), in the postcentral gyrus of the parietal lobe; the secondary somatosensory cortex (S2), in the parietal operculum; the ACC/MCC, in the cingulate gyrus; and the insula, in the lobe of the insular cortex (IC), which is found under the temporal and frontal lobes, in the Sylvian fissure.52 Methods that involve making a lesion specific to structures or recording nerve cells in these same localized regions have only allowed us to have a fragmented view of the role of the cortex in pain. We have sufficient data to conclude that cortical structures such as the SI contribute to the sensory-discriminative component of pain, whereas the frontal, cingulate, and insular cortical structures are involved in the motivational-affective components.51–53
In summary, our growing understanding of the role of the higher centers in pain allows us to realize the complex balance between the sensory and affective components. It is now easier than ever to accept the importance of the mutual influence between emotions and sensations in the pain experience. Certain higher centers (SI, SII) specialize in the sensory-discriminative component of pain to give precise information on the location, intensity, and all the other characteristics of the nociceptive stimulation. Other centers (ACC, IC) specialize in the emotional appreciation of pain. The affective component is not only associated with the intensity of the stimulation but also refers to other emotions, such as anticipation or fear.54 For example, we may experience suffering when we attend to the pain of another person, especially when this person is dear to us. A study revealed that empathy for other people’s suffering activates the same brain centers associated with the motivational-affective component of pain as if it were our own pain but without the activity of the centers associated with the sensory-discriminative component.55 Our perception of the pain of others is therefore quite real in cerebral terms!
Central sensitization refers to a phenomenon whereby the second neuron membrane permeability changes and responds at a higher frequency when recruited by nociceptive (hyperalgesia) and non-nociceptive primary input (allodynia). Central sensitization is defined as an increase of excitability and spontaneous discharge of the dorsal horn neurons with an associated increase in the receptive field of these neurons. This phenomenon will principally affect the wide dynamic range (WDR) neurons from the dorsal horn and is dependent on the activity of the N-methyl-D-aspartate (NMDA) receptors.34,56 These neurophysiological and neurochemical mechanisms involved in central sensitization are responsible for the modification of the spinal nociceptive circuitry and contribute to the maintenance of pain (Fig. 34–4).
In the spinal cord, secondary hyperalgesia is a phenomenon that refers to sensitization.57 Repeated recruitment of C fibers following an injury will produce central sensitization by changing the response properties of the membrane of secondary neurons. This will result in an increase in the firing rate, a phenomenon known as wind-up.17 The high-frequency recruitment of C fibers, either by increased repetitive stimuli or by a tonic stimulation,58 will then induce an increase in the perceived pain, even if the intensity of the stimulation remains constant. This central sensitization at the spinal level can persist for minutes, but can also be present for hours and even days.59 The prolonged activation of the NMDA receptors will induce the transcription of rapidly expressed genes (c-fos, c-jun), resulting in sensitization of nociceptors. This neuronal plasticity of the secondary neuron will result in reduced threshold and enlargement of their receptive field in the spinal cord and produce hyperalgesic and allodynic responses that may persist even after the injury is healed.
It is now well documented that several supraspinal facilitatory and inhibitory mechanisms play a major role in pain perception and most probably in certain chronic pain conditions.60 The work of Fields and colleagues describing the activation of “ON” cells and inhibition of “OFF” cells in the brainstem during nociceptive activity has demonstrated the importance of facilitatory mechanisms in amplifying the nociceptive response.61 Proglumide, an antagonist of cholecystokinin (CCK), has been shown to block nocebo hyperalgesia62; furthermore CCK directly activates “ON” cells.63 It is therefore possible that the hyperalgesia reported during the nocebo effect depends on these excitatory bulbospinal circuits that facilitate spinal nociceptive activity.
Recent studies have also demonstrated that certain physiological conditions, such as nociceptive hyperactivity, may change the usual neuronal response to specific neurotransmitters. A particular example is the hyperalgesic effect that can be observed in some patients using opioid medications.64 Therefore, drugs with opioid activity could, under certain circumstances, produce a completely opposite effect and enhance pain by producing hyperalgesic responses.64,65 The same is also true for gamma-aminobutyric acid (GABA), which has been clearly identified as an inhibitory neurotransmitter but in certain conditions may cause depolarization of neurons.66 Negative emotions, expectation, or conditioning triggered by the activity from the prefrontal cortex (ventrolateral PFC—vlPFC; ventral-medial PFC—vmPFC) will recruit descending facilitatory mechanisms from the brainstem (rostroventral medulla—RVM) that may play an important role in some chronic pain conditions.67–72
These observations support the concept of pain as a dynamic phenomenon. An understanding of these complex mechanisms can help explain the clinical variability of response to treatments in patients with chronic pain.
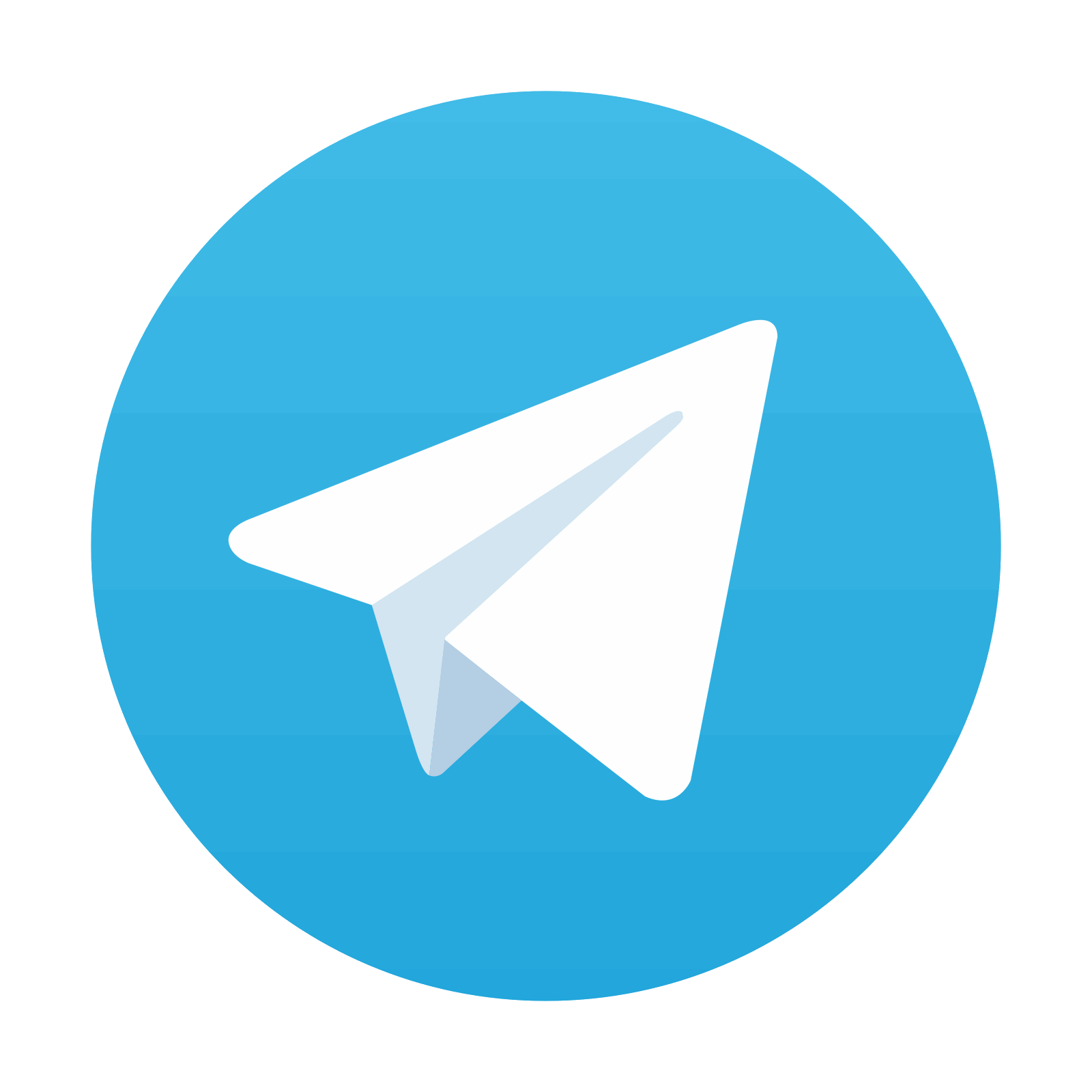
Stay updated, free articles. Join our Telegram channel

Full access? Get Clinical Tree
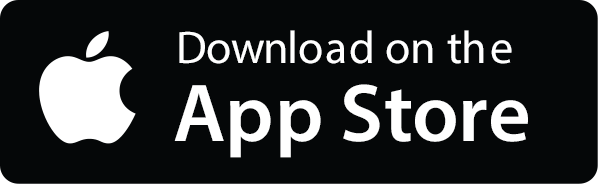
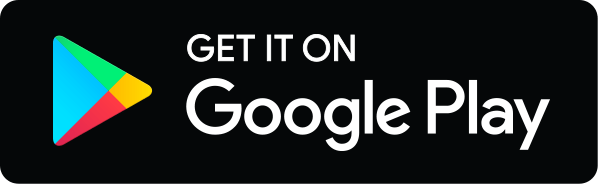