Systemic lupus erythematosus (SLE) is a complex disease characterized by numerous autoantibodies and clinical involvement in multiple organ systems. The immunologic events triggering the onset and progression of clinical manifestations have not yet been fully defined, but a central role for B cells in the pathogenesis has been brought to the fore in the last several years. The breakdown of B-cell tolerance is likely a defining and early event in the disease process and may occur by multiple pathways, including alterations in factors that affect B-cell activation thresholds, B-cell longevity, and apoptotic cell processing. Antibody-dependent and -independent mechanisms of B cells are important in SLE. Thus, autoantibodies contribute to autoimmunity by multiple mechanisms including immune complex mediated type III hypersensitivity reactions, type II antibody-dependent cytotoxicity, and by instructing innate immune cells to produce pathogenic cytokines including interferon α, tumor necrosis factor, and interleukin 1. Recent data have highlighted the critical role of toll-like receptors as a link between the innate and adaptive immune system in SLE immunopathogenesis. Given the large body of evidence implicating abnormalities in the B-cell compartment in SLE, there has been a therapeutic focus on developing interventions that target the B-cell compartment. Several different approaches to targeting B cells have been used, including B-cell depletion with monoclonal antibodies against B-cell–specific molecules, induction of negative signaling in B cells, and blocking B-cell survival and activation factors. Overall, therapies targeting B cells are beginning to show promise in the treatment of SLE and continue to elucidate the diverse roles of B cells in this complex disease.
Systemic lupus erythematosus (SLE) is a complex autoimmune disease with considerable heterogeneity in clinical manifestations and disease course, characterized by pathogenic autoantibody formation, immune complex deposition, and end-organ damage. The mortality and morbidity of patients with SLE has significantly improved during the last few decades. In the 1950s, the 4-year survival rate for lupus was approximately 50%; more recent series estimate 5-year and 10-year survival rates of 96% and 85%, respectively. Despite these improvements, SLE continues to be associated with significant morbidity and a three- to fivefold increased mortality compared with the general population. Moreover, there is a group of patients with SLE who continue to suffer from aggressive disease that does not respond to conventional treatments.
The continuing need for more effective therapies with less toxic side effects has led to the interest in targeted biologic therapies for severely affected patients who are refractory to or cannot tolerate traditional therapies. However, major obstacles in finding efficacious therapies for SLE include the challenges of clinical trial design given the low prevalence of disease, great clinical heterogeneity, relapsing-remitting course, and lack of well-established end points. Partly because of these challenges, no new drugs have been approved for the treatment of SLE in more than 50 years. Despite these difficulties there is reason for optimism, including concerted efforts toward improving lupus clinical trial methodology that have recently led to a successful outcome in 2 clinical trials of a B-cell targeted biologic in SLE. Moreover, our understanding about the pathogenesis of SLE has grown substantially in the past decade, leading to an increase in promising biologic therapies. This article reviews the state-of-the-art pathophysiology of SLE, providing the rationale for B-cell targeted therapies, followed by a critical evaluation of the efficacy and safety of B-cell depletion (BCD) with anti-CD20 monoclonal antibody therapy in the management of the disease, and other promising B-cell targeted approaches.
Role of B cells in SLE
Although multiple immunologic abnormalities are important for the development and clinical expression of SLE, a large body of evidence strongly points to the B cell as a critical player in the pathogenesis of this disease.
B-cell Tolerance Loss
As SLE is characterized by the generation of large amounts of autoantibodies directed against chromatin and various other self-antigens the loss of B-cell tolerance is believed to play a key role in the disease. Evidence that the breakdown of B-cell tolerance likely occurs early in SLE and may precede or trigger other immune abnormalities is provided by the demonstration that patients with SLE express antinuclear antibodies (ANAs) several years before the onset of clinical disease. The lag time observed between the appearance of ANAs and clinical expression of SLE may be explained by the need for epitope spreading and generation of increasingly pathogenic autoantibodies.
Numerous single gene defects affecting the B-cell compartment can lead to lupuslike disease in murine models, and many of these defects share a common end point, the loss of B-cell tolerance. At least 3 broad categories of defects that can lead to a lupus like phenotype have been defined in the mouse and are instructive for thinking about B-cell abnormalities in SLE. These defects may affect (1) B-cell activation thresholds (eg, Fc receptor [FcR]), (2) B-cell longevity (eg, B-cell activator of the tumor necrosis factor [TNF] family [BAFF] transgenics), or (3) apoptotic cell/autoantigen processing (eg, mer knockout). Although many alterations in B-cell signaling or costimulatory molecules that may alter (1) or (2) have been shown to lead to a lupus like phenotype in the mouse, their relevance for human SLE and even spontaneous murine models of SLE is not well defined. Kumar and colleagues have recently elucidated the mechanism by which the Sle1 susceptibility locus derived from lupus-prone New Zealand Mixed (NZM2410) mice contributes to the development of autoimmunity. A gene within this locus encoding a member of the signaling lymphocyte activation molecule (SLAM) family was found to be highly expressed in immature B cells and altered in these lupus-prone mice in such a way as to impair signaling and impede antigen-driven negative selection (B-cell deletion, receptor revision, and anergy induction). This finding suggests that some of the genes that contribute to lupus may function by downregulating B-cell receptor (BCR) signaling at the immature stage and impairing B-cell tolerance.
In contrast, other B-cell signaling defects may cause upregulated signaling, as exemplified by loss of inhibitory FcR function. Thus, FcγRIIb deficiency leads to a lupus like phenotype in mice, although with different penetrance depending on mouse strain. Moreover, deficiency of the inhibitory FcγRIIb reduces the threshold for autoreactive B-cell activation, and restoration of proper FcγRIIb expression on B cells in lupus-prone mice prevents the expansion and accumulation of autoantibody-producing plasma cells (PCs). These findings are even more meaningful given that polymorphisms in FcγRIIb are associated with human SLE and have a direct functional consequence on B-cell signaling. Thus, an FcγRIIb membrane spanning Ile232 to Thr232 substitution associated with lupus in Asian populations caused decreased FcR lipid raft association and greater, more sustained calcium mobilization and downstream biochemical signaling events on B-cell receptor engagement. Moreover, other defects may lead to decreased expression of FcgRIIb as recently demonstrated in human lupus memory B cells. Such alterations in B-cell signaling proteins could explain earlier observations of increased calcium responses to BCR ligation in SLE B cells. Olferiev and Crow recently approached this question in human lupus by comparing the gene-expression signatures specifically in memory B cells and found the underexpression of several inhibitory receptors including CD22 and CD72, and the overexpression of type I interferon (IFN) inducible genes. Overall, such defects in B-cell signaling pathways are important because they may contribute to the loss of peripheral B-cell tolerance in SLE.
Alterations in B-cell longevity also can lead to lupuslike phenotypes, as exemplified by transgenic expression of BAFF, a key cytokine that promotes B-cell survival. These mice develop a lupuslike phenotype with excessive numbers of mature B cells, spontaneous germinal center (GC) reactions, autoantibodies, high PC numbers, and immunoglobulin (Ig) deposition in the kidney. Moreover, lupus-prone mice have elevated levels of circulating BAFF, and administration of soluble BAFF receptor ameliorates disease progression and improves survival. The importance of BAFF in human SLE has been demonstrated by the finding of increased serum levels in patients with SLE and the correlation with serum IgG and autoantibody levels. Excessive BAFF may be another factor that promotes the survival of autoreactive B cells in the periphery. Thus, 2 recent reports have shown that BAFF is a key limiting resource for early (transitional) B-cell survival, with autoreactive B cells surviving selection into the mature pool only when BAFF was in excess. Miller and colleagues have proposed a model of B-cell selection and tolerance induction that postulates that the number of cells entering the transitional B-cell pool must exceed the available space if appropriately stringent negative selection is to occur. Events that compromise this rule, including a decrease in incoming cell numbers or increases in BAFF, may circumvent negative selection of autoreactivity ( Fig. 1 ).

The impaired clearance of apoptotic debris may also lead to SLE and may do so in part by providing large amounts of self-antigen and immune complexes that deliver stimulatory signals to autoreactive B cells. Several publications in recent years indicate that such apoptotic blebs and immune complexes contain ligands for toll-like receptors (TLR), including RNA or DNA, which can provide costimulatory signals for autoreactive B cells, as further described later.
Abnormalities in the B-cell Compartment in Human SLE
Recent work has demonstrated the role of the breakdown of peripheral B-cell tolerance mechanisms in human SLE. Thus, Pugh-Bernard and colleagues have shown that an important tolerance checkpoint operates in healthy subjects to censor autoreactive (9G4) B cells in the mature naive compartment, thereby preventing the expansion of these cells into the memory compartment, a checkpoint recently corroborated by others and further shown by the authors to be faulty in SLE. Other work by Wardemann and colleagues has shown that 50% to 75% of newly produced human B cells are autoreactive and must be silenced by tolerance mechanisms. Key checkpoints to censor autoreactive B-cell clones occur at the immature B-cell stage in the bone marrow (BM) and between new transitional emigrants and mature B cells in the periphery. In this system up to 20% of mature naive B cells were self-reactive, indicating the need for additional censoring mechanisms at this stage, as suggested by Pugh-Bernard and colleagues and recently shown in the transition to the IgM memory compartment. Wardemann and colleagues have also studied a small number of patients with SLE (n = 3) and found the transitional B-cell checkpoint to be defective although with some heterogeneity, with 41% to 50% of antibodies from the mature naive compartment retaining autoreactivity with HEp-2 cell lysates. These data are intriguing, although the precise point of tolerance breakdown, the variability in this finding in different patient subsets, and the cause of this tolerance checkpoint defect remain to be defined.
A large body of additional evidence indicates that B cells are abnormal in human SLE, with defects ranging from increased calcium flux on signaling through the BCR to high or aberrant expression of costimulatory molecules such as CD80, CD86, and CD40 ligand. B-cell alterations in SLE peripheral blood seem to be more prominent and fluid than in other autoimmune diseases. A range of abnormalities in B-cell homeostasis in SLE have been observed, including a naive B-cell lymphopenia, expansion of peripheral blood plasma cells, increased transitional B cells, and expansion of activated memory B-cell subsets. The authors surmise that this reflects the systemic nature of the autoimmune process with frequent cycles of activation, differentiation, and traffic between secondary lymphoid organs and target tissues. The frequency and absolute number of PCs in peripheral blood has correlated with disease activity and antidouble-stranded DNA (anti-dsDNA) titers. More recent studies have begun to refine subsets of PCs, including a human leukocyte antigen (HLA) dopamine receptor high fraction, that may better delineate plasmablasts enriched for autoreactivity and correlating with disease activity. Similarly, the authors’ group has elucidated the heterogeneity that exists in human B-cell memory and found that active patients with SLE have an expansion of effector memory B-cell populations positive for markers of homing to sites of systemic inflammation (CXCR3+) and negative for lymphoid homing markers (CD62L−). Other groups have found a subset of CD27-IgD-CD95+ memory B cells with an activated phenotype that correlated with disease activity and serologic abnormality. Abnormal expansion of peripheral blood B cells with a pre-GC phenotype has also been reported and proposed to indicate exuberant or abnormally regulated GC reactions in SLE. However, in many cases these cells may actually represent immature transitional B cells as opposed to pre-GC cells.
Overall, the frequency of these diverse B-cell subset abnormalities, their association with each other and other immunologic abnormalities, and relationship with disease activity and disease subsets remain to be better elucidated in larger longitudinal cohorts of patients with SLE. However, several of the B-cell targeted therapies have been shown to reverse at least some of these peripheral B-cell abnormalities. For example, the authors have shown that CD20 targeted BCD normalizes naive lymphopenia, effector memory B-cell expansion (CD27-IgD- double-negative B cells), the presence of PC precursors, and expansion of autoreactive memory B-cell populations. In other studies, BAFF blockade has also been shown to alter peripheral B-cell homeostasis in SLE with decreases in transitional B cells, naive B cells, and plasmablasts, but with less significant effects on the memory B-cell compartment at least at early time points.
The Diverse Role of B Cells in the Initiation and Propagation of Autoimmunity
B cells may participate in the immune dysregulation of SLE at multiple levels by: (1) serving as the precursors of antibody-secreting cells, (2) taking up and presenting autoantigens to T cells, (3) helping to regulate and organize inflammatory responses through cytokine and chemokine secretion (such as interleukin 10 [IL-10], IL-6, IFN-γ, and lymphotoxin-α), and (4) regulating other immune cells (5) ( Fig. 2 ). The importance of these latter functions has been demonstrated in murine SLE, in which B cells have been found to be critical to the development of disease even when they are unable to secrete autoantibodies. Thus, genetically B-cell deficient J H knockout MRL/lpr lupus-prone mice have strikingly attenuated disease, with the expected absence of autoantibodies but also the surprising lack of T-cell activation. Chan and colleagues used a novel approach to further elucidate the role of B cells in SLE by generating MRL/lpr mice that express a mutant transgene encoding surface Ig that cannot be secreted. These mice have B cells, but no circulating Ig, yet develop T-cell activation and nephritis. This landmark study was the first to highlight that B cells can play a pathogenic role in lupus independent of serum autoantibody and has been recently supported by the authors’ observations that BCD with anti-CD20 has robust effects in the treatment of murine lupus without changes in autoantibodies. This notion has also been corroborated in humans by the authors’ own and others’ findings that clinical improvement in patients with SLE treated with rituximab correlates with BCD and precedes by several months any decline in serum levels of relevant autoantibodies.

Autoantibody-independent Functions for B Cells in SLE
There has been much speculation about what the key autoantibody-independent functions of B cells are in SLE ( Fig. 2 ). A particularly novel function may be direct effects on lymphoid neogenesis through the production of lymphotoxin α (LTα). The formation of tertiary or ectopic lymphoid tissue formation is a process that may lead to dysregulated B-/T-cell interactions and local amplification of autoimmune responses in multiple autoimmune diseases, including rheumatoid arthritis (RA), Sjögren syndrome, type I diabetes, multiple sclerosis, inflammatory bowel disease, Hashimoto thyroditis, and SLE. For example, recent reports suggest the importance of this process in human lupus nephritis (LN).
Moreover, B cells can produce numerous other cytokines and may do so in a polarized fashion, mimicking Th1/Th2 cells. So-called effector B cells (Be1 and Be2) can participate in feedback regulation of T helper cells, although the relevance of these mechanisms for SLE have yet to be demonstrated. Along similar lines, B cells play a key role in the recruitment of CXCR5+ follicular T helper (T FH ) cells to the germinal center. T FH cells provide critical assistance for follicular and GC B cells, inducing activation, differentiation, and antibody production. Recent data indicate that T FH cells and a newly defined population of extrafollicular helper T cells are dependent on B cells for development. The influence of B cells on T FH cells via ICOSL and OX40L costimulation may be important in SLE as excessive activity of T FH cells induces hyperactive GC, breakdown of B-cell tolerance, autoantibody production, and a lupuslike phenoytype in sanroque mice.
From an autoimmunity standpoint, B cells may either stimulate or inhibit pathogenic responses ( Table 1 ). Thus, evidence is accumulating for regulatory B cells capable of preventing or suppressing autoimmunity in different mouse models. This protective role can be mediated by inducing T-cell anergy during antigen presentation or inducing Treg expansion or activity. B cells may also directly suppress Th1- and Th17-mediated diseases. These activities are mediated, at least in part, by the production of IL-10 or transforming growth factor β (TGF-β) and may control various autoinflammatory diseases, including inflammatory arthritis, inflammatory bowel disease, autoimmune diabetes, experimental autoimmune encephalitis, and contact hypersensitivity. Understanding the imbalance between these opposing B-cell functions in disease and how this imbalance may be restored after targeted biologic therapy is an important area of ongoing research.
Induce Autoimmunity | Suppress Autoimmunity |
---|---|
|
|
|
|
|
|
|
|
|
|
|
|
The Interface Between Innate and Adaptive Immunity: Autoantibodies, IFN, and TLR Activation
Once B-cell tolerance is broken, autoantibodies can contribute to disease pathogenesis in SLE through several classic effector roles, including the formation of damaging immune complexes (immune complex mediated type III hypersensitivity reactions) and direct pathogenesis (type II antibody-dependent cytotoxicity) ( Fig. 2 ). However, as alluded to earlier, evidence has emerged that autoantibodies can play an active role in propagating the autoimmune process in SLE, through immune cell activation involving RNA- or DNA-containing autoantigens and TLRs. Plasmacytoid dendritic cells (DCs) may be activated by costimulation of TLRs (TLR-7, -8, or -9) and FcRs via immune complex binding, stimulating the secretion of large quantities of IFN-α, a cytokine with important immunomodulatory functions that include activation and maturation of DCs and stimulation of T and B cells. In combination with TLR-7 and -9 activation of myeloid DCs to produce BAFF, a feedback loop is generated that triggers more B-cell activation ( Fig. 1 ). Moreover, by binding to cell surface autoantibodies and subsequently to TLR-7 or -9 within B cells, RNA- or DNA-containing autoantigens or immune complexes can directly trigger activation and proliferation of autoantibody-producing B cells. In support of the importance of TLR signaling to lupus disease pathogenesis and B-cell activation, deficiency of TLR-7 or -9 can prevent autoantibody production in various mouse models. It was recently shown that the murine autoimmune Yaa locus represents a TLR-7 duplication that increases the responsiveness of B cells to TLR-7 ligands, thus contributing to a break in B-cell tolerance. One of the mechanisms by which antimalarials likely exert their effect in SLE is via inhibition of TLR signaling.
As reviewed elsewhere, recent evidence suggests a prominent role for IFN-α activation in SLE. IFN-α may contribute to B-cell abnormalities in SLE, promoting the differentiation of activated B cells into plasmablasts and in conjunction with TLR stimulation triggering B-cell expansion and a lowered activation threshold for autoreactive B cells. The authors have also recently reported a novel role for IFN-α activation in the BM of patients with SLE by decreasing B-cell lymphopoeisis, contributing to B-cell lymphopenia, and theoretically decreasing the stringency of B-cell negative selection.
Although the relative importance of autoantibody-dependent versus -independent roles of B cells in human SLE pathogenesis remains to be defined, given the multiple pathogenic roles of autoantibodies in SLE enumerated earlier, it could be postulated that the most effective therapeutic interventions might decrease autoantibody levels, which may require targeting of the PC compartment directly or indirectly. To do so effectively, it is important to understand the nuances of PC biology. In particular, the premise that autoreactive PCs are short-lived and continually replenished from ongoing immune responses has recently been called into question. There is accumulating evidence that some autoreactive PC populations may be long-lived and resistant to conventional therapies.
Therapeutic targeting of the B-cell compartment
Several different approaches to targeting B cells have been used: (1) BCD with monoclonal antibodies against B-cell–specific molecules (eg, anti-CD20), (2) induction of negative signaling in B cells (eg, anti-CD22); (3) blocking B-cell survival and activation factors (eg, anti-BAFF), and (4) blocking costimulatory interactions between B and T cells. Many of these agents are currently undergoing formal testing in clinical trials or are under development ( Table 2 ).
Compound | Description | Stage of Development |
---|---|---|
BCD | ||
| Chimeric anti-CD20 monoclonal antibody | Phase III SLE |
| Humanized anti-CD20 monoclonal antibody | Phase III SLE |
| Anti-CD20 SMIP (small modular immunopharmaceutical) | Phase III RA |
| Fully human anti-CD20 monoclonal antibody | Phase III RA |
Inhibitory signaling | ||
| Humanized anti-CD22 monoclonal antibody | Phase II in SLE |
Costimulatory blockade | ||
| Monoclonal antibody against CD40L | No longer in development |
| Fusion protein of CTLA4: blocks B7-CD28 costimulation | Phase II SLE |
Cytokine blockade | ||
| Fully human anti-BAFF monoclonal antibody | Advanced development in SLE |
| Fusion protein of the BR3 BAFF receptor | Phase II RA |
| Fully human antibody against BR3 | Phase II RA |
| Fusion protein of the TACI receptor blocks BAFF/APRIL | Phase I, II RA, SLE |
Therapeutic targeting of the B-cell compartment
Several different approaches to targeting B cells have been used: (1) BCD with monoclonal antibodies against B-cell–specific molecules (eg, anti-CD20), (2) induction of negative signaling in B cells (eg, anti-CD22); (3) blocking B-cell survival and activation factors (eg, anti-BAFF), and (4) blocking costimulatory interactions between B and T cells. Many of these agents are currently undergoing formal testing in clinical trials or are under development ( Table 2 ).
Compound | Description | Stage of Development |
---|---|---|
BCD | ||
| Chimeric anti-CD20 monoclonal antibody | Phase III SLE |
| Humanized anti-CD20 monoclonal antibody | Phase III SLE |
| Anti-CD20 SMIP (small modular immunopharmaceutical) | Phase III RA |
| Fully human anti-CD20 monoclonal antibody | Phase III RA |
Inhibitory signaling | ||
| Humanized anti-CD22 monoclonal antibody | Phase II in SLE |
Costimulatory blockade | ||
| Monoclonal antibody against CD40L | No longer in development |
| Fusion protein of CTLA4: blocks B7-CD28 costimulation | Phase II SLE |
Cytokine blockade | ||
| Fully human anti-BAFF monoclonal antibody | Advanced development in SLE |
| Fusion protein of the BR3 BAFF receptor | Phase II RA |
| Fully human antibody against BR3 | Phase II RA |
| Fusion protein of the TACI receptor blocks BAFF/APRIL | Phase I, II RA, SLE |
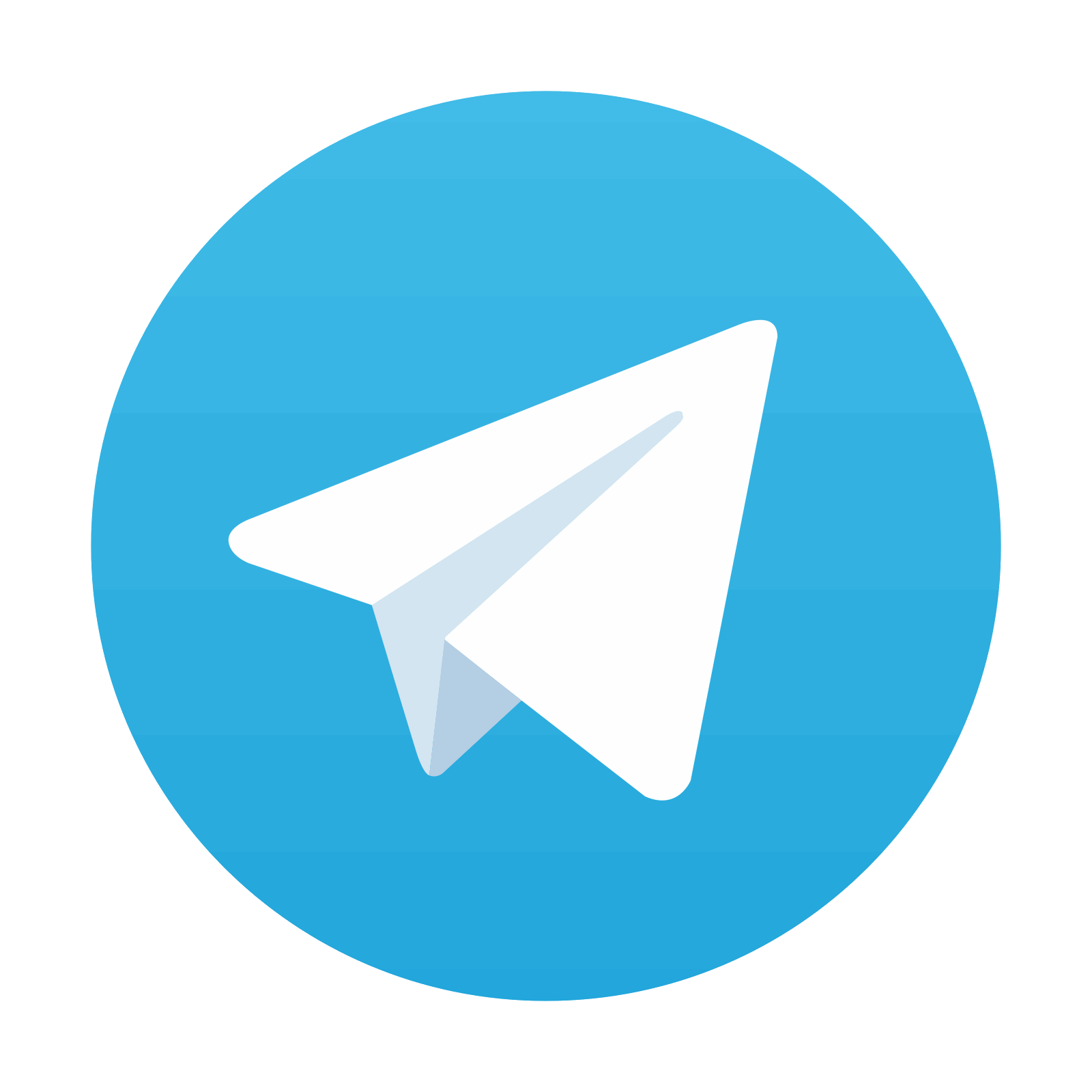
Stay updated, free articles. Join our Telegram channel

Full access? Get Clinical Tree
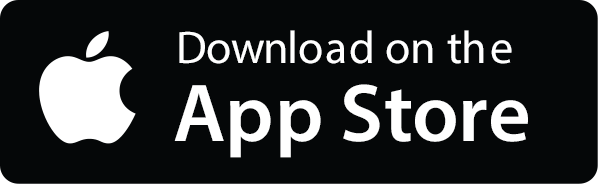
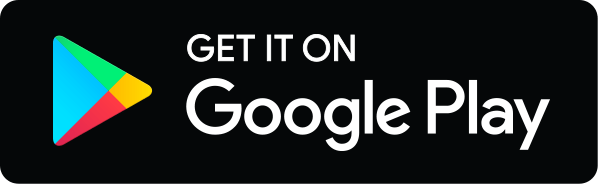
