Introduction
The delivery of cell- and non-cell-based therapies can generally be classified as either autologous or allogenic. Autologous treatments involve a procedure in which the patient’s own tissues are collected and subsequently reintroduced into the patient’s own body. Some benefits of using autologous products over allografts include the innate decrease in immunologic side effects and tissue rejection, transfusion reaction, and reduced possibility of disease transmission. Autologous products include platelet-rich plasma (PRP), platelet lysate (PL), alpha-2 macroglobulin, interleukin-1 (IL-1) receptor antagonist, bone marrow aspirate (BMA) and concentrate, adipose tissue, and cultured cellular injectates.
Platelet-Rich Plasma
PRP is an autologous preparation of concentrated platelets isolated from whole blood by centrifugation, defined as having a plasma product with total platelet count greater than normal physiologic levels. PRP therapy is currently being used in musculoskeletal medicine as an injectable therapy for bone, joint, and connective tissue pathology. Platelets are an abundant source of growth factors that when released are available to aid in tissue repair. While the science behind PRP continues to evolve, there is a need for optimizing and characterizing PRP formulations.
PRP is formulated using various amounts of whole blood, with the final volume being around 10% of the original whole blood volume. The general process is outlined below, though it should be noted that each commercial kit will have differing protocols, including starting volume, varying speeds of centrifugation, and duration of the spin protocol, among others. There are at least 33 commercial PRP systems available on the market at the time of publication of this textbook. , Each system varies in collecting tubes utilized, centrifugation speed, single versus double spin, use (or lack) of activating agents, leukocyte and red blood cell inclusion, and final platelet volume and total number. There is significant variability across commercial kits. A 2017 study assessed platelet count, red blood cell count (RBC), leukocyte count, pH, and glucose in four commercially available PRP kits. The study revealed variation in platelet and other blood component concentrations, and lack of standardization of PRP preparation between kits. Proponents of these systems cite internal consistency in platelet concentration and ease of use as reasons to use them; however, laboratory protocol can also produce precise PRP formulations without specialized commercial equipment.
Another variable in PRP preparation is the use of an exogenous activator. While used more commonly in surgical applications, these activators promote platelet clotting for surgical scaffold applications, degranulation, and subsequent growth factor release. Exogenous activators are commonly calcium (i.e., calcium chloride and calcium gluconate) or thrombin based. The majority of reported studies in the literature use 10% calcium chloride. Thrombin and arachidonic acid are other activators that have been used. There has been increasing interest in activator selection as some in vivo studies have found that they may variably release growth factors. Exogenous activator may extend the growth factor release period to 7 to 10 days beyond the initial injection, in addition to the platelet clot providing a surgical scaffold. , A more detailed discussion of scaffolding applications is beyond the scope of this chapter.
Given the diversity in PRP preparation systems and formulations, characterizing PRP is important to optimizing formulations and interpreting the literature. There have been more than 10 proposed classifications for PRP since 1999. , Most call for characterization of platelet count, presence of cell lines besides platelets, and nuances of preparation such as the use of an exogenous activator. For example, the PAW system is based on absolute P latelet count, method of platelet A ctivation, and presence or absence of W hite blood cells (WBCs). The PLRA classification by Mautner and colleagues. was one of the first classification systems to expand its characterization by including red blood cell and white blood cell counts. Newer classification systems such as MARSPILL aim to address parameters such as concentration folds over baseline, amount of spins, and spin speed, but classification systems have not caught on widely due to the fragmented nature of PRP research. ,
There are ongoing efforts to optimize PRP formulations, with a focus on platelet concentration. Current commercial systems vary widely in their platelet concentrations, ranging from 0.52 to 9× the baseline in some studies. It is important to note that the positive effects of PRP can be dose dependent, and proliferation of tendon stem/progenitor cells cultured in various concentration of PRP has been shown to increase in a dose-dependent manner in up to a 10% PRP dose. At high concentrations, PRP may begin to exhibit anti-chondrogenic activity and impair healing. , However this is largely dependent on which growth factors are being degranulated, i.e., chondrotoxic or chondroprotective mediators and may not be platelet concentration dependent. Conversely, a 2019 study suggests a dose-dependent response to higher PL concentrations in promoting tenocyte proliferation in vitro in the aged population (>50 years old) but not young donors .
The presence and absence of leukocytes in PRP is another common area of discussion. Leukocyte infiltration of tissues has been linked to both muscle injury and repair and, as such, the argument can be made for or against included leukocytes in PRP formulations. Leukocyte-poor (LP) PRP is generally defined as any PRP formulation containing fewer WBCs than baseline and leukocyte-rich (LR) PRP conversely contains more WBCs than baseline.
Mechanism of Action and Clinical Applications
Currently, there are no guidelines on PRP dosing and the use of specific formulations for different pathologies. However, some studies have reported LP-PRP may be beneficial for cartilaginous injuries and LR-PRP for tendinous injuries. However, other studies report that LR-PRP should be avoided in osteoarthritis and tendinopathies because WBCs release proteases and reactive oxygen species that may harm remaining cartilage or tendinous tissue, respectively. One study noted that compared to treatment with LP-PRP and phosphate-buffered saline (PBS), synovial cells treated with LR-PRP resulted in significantly greater cell death and some proinflammatory mediator production. While the exact dosing and preparation are unknown, some studies have recommended that the platelet concentration for knee osteoarthritis and tendinopathies should target close to 3 to 4× and be leukocyte free. ,
There is no clear in vivo mechanism for the therapeutic effects of PRP and its subtypes; however, PRP’s therapeutic effects are often attributed to bioactive factors and high concentration of growth factors. The true biologic response to PRP is poorly understood and likely pleiotropic, and can have positive benefits on one tissue and deleterious effects on other. In vitro, platelets play important roles in wound healing including re-epithelialization, granulation tissue formation, cartilage and bone matrix formation/remodeling, chemotaxis, and angiogenesis. , Platelets accomplish this through their alpha granules that contain the necessary growth factors for these processes, such as platelet-derived growth factor (PDGF), vascular endothelial growth factor (VEGF), epidermal growth factor (EGF), and fibroblast growth factor-2 (FGF-2) ( Table 7.1 ). They also produce tissue growth factor-β1 (TGF-β1)—one of the most important mediators in connective tissue regeneration and potent inhibitors of matrix metalloproteinases (MMP-1, -3, -9), which inhibit collagen synthesis.
Reproduced with permission from Regenerative Medicine, An Issue of Physical Medicine and Rehabilitation Clinics of North America (Volume 27-4) ( The Clinics: Orthopedics (Volume 27-4)). 1st Edition. Santos F. Martinez: Elsevier 2016
Data from Refs.
Malanga GA, Goldin M. PRP: review of the current evidence for musculoskeletal conditions. Curr Phys Med Rehabil Rep . 2014;2:1–5.
Davis VL, Abukabda AB, Radio NM, et al. Platelet-rich preparations to improve healing. Part I: workable options for every size practice. J Oral Implantol . 2014;40(4):500–510.
Boswell SG, Cole BJ, Sundman EA, et al. Platelet-rich plasma: a milieu of bioactive factors. Arthroscopy . 2012;28(3):429–439.
Blair P, Flaumenhaft R. Platelet alpha-granules: basic biology and clinical correlates. Blood Rev . 2009:23(4):177–189.
Growth Factor | Function |
---|---|
PDGF | Stimulates cell proliferation, chemotaxis, and differentiation Stimulates angiogenesis |
TGF-β | Stimulates production of collagen type I and type III, angiogenesis, re-epithelialization, and synthesis of protease inhibitors to inhibit collagen breakdown |
VEGF | Stimulates angiogenesis by regulating endothelial cell proliferation and migration |
EGF | Influences cell proliferation and cytoprotection Accelerates re-epithelialization Increases tensile strength in wounds Facilitates organization of granulation tissue |
bFGF | Stimulates angiogenesis Promotes stem cell differentiation and cell proliferation Promotes collagen production and tissue repair |
IGF-1 | Regulates cell proliferation and differentiation Influences matrix secretion from osteoblasts and production of proteoglycan, collagen, and other noncollagen proteins |
It is proposed that growth factors from PRP induce processes of inflammation and cell recruitment, angiogenesis, and matrix formation by delivering a large quantity of platelets to the site of injury; however, this is still the subject of ongoing research. It is important to note that PRP’s effects through a complex sequence of growth factor communication within the tissue microenvironment facilitates healing of the remaining tissue and does not regenerate absent tissue.
Common clinical applications for PRP use include, but are not limited to: tendinopathies (patellar, rotator cuff, gluteal, lateral epicondylitis, plantar fasciitis), ligamentous tears (ulnar collateral ligament, anterior cruciate ligament [ACL]), muscular strains (hamstring, gastrocnemius, lumbar multifidus), peripheral mononeuropathies (carpal tunnel syndrome), spine pathology (sacroiliitis, facet arthropathy, degenerative disc disease, and radiculopathy), and peripheral joints (knee osteoarthritis, carpometacarpal joint pain, and temporomandibular joint syndrome).
Data on Efficacy
The main limitation for clinical use of PRP is not a lack of data but rather heterogeneous data. There is a large variation in standardization in its preparation and delivery, making it difficult to discern potential beneficial effects of PRP. For instance, while PRP has demonstrated overall favorable outcomes in some systematic reviews, the studies had varying methods of PRP preparation, patient populations, and stages of disease.
Shen and colleagues conducted a systematic review that found that most patients had significantly improved pain relief and self-reported function after PRP injection for knee osteoarthritis compared to saline placebos, hyaluronic acid (HA), ozone, and corticosteroid injections. It was also reported that 10 of the 14 included studies had high risk of bias associated with them, mostly due to lack of investigator blinding. Laver and colleagues published a recent systematic review of PRP in knee and hip osteoarthritis encompassing 29 studies, including 1 hip and 8 knee randomized controlled trials (RCTs) and 11 total studies controlled with HA. For the studies controlled with HA, 9 of 11 studies found that PRP was significantly superior to HA in terms of symptom improvement. In general, beneficial effects of PRP therapy in osteoarthritis have been more effective in cohorts with milder disease and younger populations. ,
A 2017 systematic review and meta-analysis comprised exclusively from Level 1 RCTs in rotator cuff injuries and lateral epicondylitis reports significantly less pain in PRP-treated groups compared to control in the long term. This differs from prior meta-analysis, largely including low-powered studies and heterogenicity in comparator outcomes, which have published contrasting results.
A double-blinded RCT by Dragoo and colleagues compared the clinical outcomes in patients with patellar tendinopathy after a single ultrasound-guided, leukocyte-rich PRP injection with dry needling compared to dry needling in 23 patients. They reported that while the regimen of standardized eccentric exercise and PRP injection with dry needling appeared to help patients with patellar tendinopathy more than dry needling alone at 12 weeks ( P = .02) as evaluated by the Victorian Institute of Sports Assessment (VISA), the benefits of PRP was not long lasting ≥26 weeks ( P = .66). An RCT of a single injection of LR-PRP or LP-PRP was not found to be more effective than saline for the improvement of patellar tendinopathy symptoms in a study by Scott and colleagues. There was no significant difference in the VISA score, pain during activity, or global rating of change at 12 weeks, 6 months, and 12 months. A systematic review and meta-analysis of nonsurgical treatments of patellar tendinopathy by Andriolo and colleagues noted that while eccentric exercises seem to be the most beneficial with short-term follow-ups less than 6 months ( P < .05), multiple PRP injections may offer more satisfactory results at long-term follow-up (≥6 months, P < .05).
Wu and colleagues conducted a single-blind RCT to assess the 6-month effect of PRP in patients with mild to moderate carpal tunnel syndrome (CTS). Sixty patients with unilateral CTS were randomized into two groups of 30, with one group receiving one dose of 3mL of PRP using ultrasound guidance and the control group receiving a night splint. The patients who received PRP exhibited a significant reduction in the visual analog scale (VAS), Boston Carpal Tunnel Syndrome Questionnaire score, and cross-sectional area of the median compared to those of the control group 6 months post-treatment ( P < .05).
A systematic review and meta-analysis by Sheth and colleagues comparing PRP to control therapies (such as physiotherapy or placebo injections) in acute muscle injuries (≤7 days) found that while the use of PRP did not significantly decrease the rate of injury at 6 months follow-up, there was significant decrease in return to play times except with a subgroup with grade 1 or 2 hamstring muscle strain. A literature review by Setayesh and colleagues on the treatment of muscle injuries with PRP found that while there were numerous clinical case series demonstrating faster healing, less swelling, and quicker return to play times for patients with muscles strains who received PRP, most studies were retrospective and few RCTs demonstrate a clear clinical benefit. They also observed variability in the injectant preparation, the platelet concentration, the presence of leukocytes, the volume of PRP injected, and the timing of treatments.
Figueroa and colleagues conducted a systematic review of prospective cohort studies or RCTs on PRP compared to control in the treatment of ACL tears, assessing graft-to-bone healing, graft maturation, and/or clinical outcomes. While they found that more studies had evidence of faster graft maturation, it appears there were mixed results, with overall findings leaning to no significant improvement in graft-to-bone healing or clinical outcomes.
Overall, there is currently a need for systematic characterization of PRP therapy, which along with appropriately conducted prospective trials, could better understand PRP’s efficacy and formulations.
Caveats/Side Effects
PRP has demonstrated an excellent safety profile with no reported major adverse events or reactions. The side effects are similar to those for any intra- or extraarticular injection, such as infection, pain, bleeding, and injury to nearby blood vessels or nerves.
Drug interactions with PRP have not been well studied and documented in literature. Nonsteroidal antiinflammatory drugs (NSAIDs), such as aspirin and naproxen, have been shown to interfere with growth factor release given the direct inhibition of cyclooxygenase pathway. , Although there is no research correlating growth factor release and efficacy of PRP treatment in vivo, these studies suggest NSAIDs and aspirin should be held prior to PRP, and other analgesics, such as acetaminophen, should be prescribed for post-injection pain control.
US Food and Drug Administration (FDA) Regulations
PRP is regulated under the FDA Center for Biologics Evaluation and Research (CBER), which exempts it from the traditional regulatory pathway. The 510(k) application for “substantially equivalent” devices is used to bring PRP products to market. There are a myriad of FDA-approved devices ranging from traditional, injectable PRP to PRP-based biomaterials intended for enhancing bone graft healing. Most of the 33 systems on the market have FDA 510(k) clearance to enhance bone graft preparations with PRP. Although PRP injections are considered off-label use, physicians are allowed to administer them under CBER as long as they are “well informed about the product…base its use on firm scientific rationale and on sound medical evidence, and… maintain records of the product’s use and effects.”
The World Anti-Doping Agency (WADA), an international independent agency that regulates doping-free sport, had prohibited PRP in 2010, but removed it from the list in 2011. The initial concern from the experts was that growth factors in PRP may stimulate muscle growth beyond the normal physiologic state and give individuals an unfair advantage; however, the prohibition was lifted as there was limited evidence for a systemic ergogenic effect of PRP. PRP appears to trigger an increase in circulating growth factors systemically, including elevated serum insulin-like growth factor-1 (IGF-1), VEGF, and basic fibroblast growth factor (bFGF), which may be banned by governing agencies, and should be used with caution in athletes as this may cause false negatives in doping tests.
Conclusion
PRP continues to be a promising therapy, especially in early osteoarthritis- and tendinopathy-related injuries. However, its use is currently limited by mechanistic understanding, cost burden, a lack of standardization in its formulation protocols, and calls for more robust blinded studies.
Platelet Lysate
PL is a cell- and platelet-free supernatant rich in growth factors and cytokines, created when the platelet membranes have been lysed, releasing intracellular proteins. Anecdotally, this is thought to have inherent antiinflammatory properties.
PL became of increasing interest in the 1980s as the growth-promoting effects of PL became studied in multiple clinical applications including wound and soft tissue injury, ocular disorders, and equine musculoskeletal injuries, and more recently in human musculoskeletal conditions. , Its main advantages include that it can be stored in a freezer and used for future or consecutive applications. Studies with in vitro and animal models demonstrate PL promote the proliferation of various cell types, including equine mesenchymal stem cells (MSCs) and tenocytes, chondrocytes, keratinocytes, osteoblasts, and bone marrow mesenchymal cells. ,
PL is derived from a platelet concentrate by mechanical disruption of PRP by freezing and thawing followed by centrifugation to separate platelet debris. There is no consensus protocol for preparation of PL. One reported protocol included freezing a PRP sample to −80°C for 30 minutes to lyse the platelets and release the growth factors. After thawing to 37°C in the water bath, the lysate is centrifuged at 1600× g for 10 minutes to separate the cell debris. Other protocols call for repeating of the freeze-thaw cycle for higher yields.
Mechanism of Action and Clinical Applications
The mechanism of action of PL is poorly understood; however, theoretically, the mechanism of action and clinical effectiveness should be similar to PRP. There are no clear indications for the use of PL, but case reports and case series have reported the use of PL for knee osteoarthritis, lumbar radiculopathy, and lateral elbow tendinopathy. , , It has been theorized that PL may be of more benefit around nerves as it is thought to be more antiinflammatory than PRP. However, this has not been studied in detail and there is no published literature on the effect of PL on nerve injuries outside of the Centeno and colleagues’ lumbar radiculopathy study mentioned above.
Data on Efficacy
There are limited data regarding clinical efficacy of PL. In a prospective open-label study of 48 patients, Al-Ajlouni and colleagues suggests intra-articular (IA) injection of autologous PL in patients with osteoarthritis of the knee is effective in reducing pain and restoring function without provoking local or systemic adverse events. This is consistent with the in vitro study in 2017 that suggested PL induces quiescent cartilage cell activation and proliferation leading to new cartilage formation. In 2017 Centeno and colleagues suggested in a retrospective review of 470 patients that PL decreases pain in lumbar radiculopathy with a significantly improvement in pain scores up to 2 years post-injection. Tan and colleagues observed in a prospective study of 56 patients significant improvement in VAS score and Mayo score for function following PL in chronic lateral epicondylitis who failed conservative therapy. There are limited data regarding clinical efficacy of PL, or in vivo study of PL on human cells.
Caveats/Side Effects
Studies on clinical efficacy of PL are limited to small case series or case reports, and the study results have not been repeated. PL as an autologous therapy has similar complications and side effects to PRP. A more comprehensive review of drugs and their effects of PL remains to be conducted, but it is possible aspirin and NSAIDs can cause an inhibitory effect on growth factor release, and therefore should be withheld in the days prior and after injection.
FDA Regulations
The use of PL is not FDA approved, however, its off-label use may be allowed by regulatory exemption per Title 21 United States Code of Federal Regulations Part 1271 (21 CFR 1271) and the 361-product exemption. In the author’s opinion, PL is exempt as it is derived from an autologous source, has a homologous use of tissue, and is processed with minimal manipulation.
Alpha-2 Macroglobulin
Alpha-2 macroglobulin protein (A2M) is a protease inhibitor, produced by the liver, and to a lesser extent by chondrocytes and synoviocytes. A2M can be found throughout the body but is greatest in the blood where it binds to and inactivates a wide variety of proteins and cytokines throughout the body. Its orthobiologic activity involves binding to proinflammatory and catabolic proteins to make a more favorable healing environment.
A2M can also be derived from autologous whole blood. Utilizing a centrifuge process similar to PRP, the platelet-poor concentrate is then used to create a concentrated volume of A2M. There are various commercial systems that can be used to purify A2M concentrate.
Mechanism of Action and Clinical Applications
In the IA environment, A2M inhibits endoproteases, particularly matrix metalloproteinases, responsible for catabolic activity. Although A2M is found in the serum, A2M is a large molecule and does not cross into synovial fluid and joint spaces at large concentrations for significant IA effect. Injection of autologous A2M concentrate, such as Cytonics APIC System, into the joint allows for the in vivo delivery of high-volume A2M, which can bind and inhibit catabolic enzymes, inhibit the inflammatory cascade, and create a favorable healing environment in the joint or other area of tissue pathology.
Data on Efficacy
Although used clinically, A2M has not been extensively studied and to date there is minimal in vivo human studies. Of the available studies, most have involved assessing osteoarthritis of the knee in preclinical models. Elevated levels of catabolic proteases and cytokines in synovial fluid have been shown to induce chondrocyte death and cartilage matrix degeneration. In a rat model of osteoarthritis induced by ACL transection, IA A2M provided chondral protection and treated knees demonstrated less cartilage damage compared to controls. , There is also suggestion in a rabbit model that it may help in healing of a ruptured ACL. Theoretically, since A2M is thought to be antiinflammatory, it is thought to potentially have utility in patients with increased joint inflammation or inflammatory arthropathies. An in vivo study by Cuellar suggests there may be efficacious use of A2M in discogenic pain in patients positive for the fibronectin-aggrecan complex (a protein biomarker of disc disease), as measured by VAS and Oswestry Disability Index (ODI) scores at 6 months following an intradiscal injection.
Caveats/Side Effects
There are limited data on clinical efficacy and there are no known specific risks of A2M other than general adverse procedural risks.
FDA Regulations
A2M is not FDA approved; however, its off-label use may be allowed by regulatory exemption per 21 CFR 1271 and the 361-product exemption. In the author’s opinion, current products to concentrate A2M from whole blood undergo “minimal manipulation” and therefore would be exempt.
Interleukin-1 Receptor Antagonist Protein
IL-1 is a proinflammatory cytokine, produced in response to injury. IL-1 causes tissue degeneration and upregulates inflammatory mediators that can result in pain. Elevated levels of IL-1 has long been associated with inflammation in rheumatologic disease and osteoarthritis, and IA levels of IL-1 correlates with osteoarthritis (OA) severity. IL-1 action is mediated by the interleukin-1 receptor antagonist (IL-1Ra) protein, or IRAP, making IRAP an attractive therapy for mediating pain and potentially modifying disease.
IL-1Ra can be obtained in two ways: (1) derived from autologous whole blood using commercial systems (Orthokine, Regenokine or Arthrex IRAP II System) or specific lab processing protocol; or (2) commercially available recombinant proteins. Recombinant forms of IL-1Ra have been FDA approved for the treatment of rheumatoid arthritis and are marketed as the drug anakinra (Kineret) and administered as daily subcutaneous injections. Autologous processing uses specialized equipment to process autologous whole blood. Glass beads bind monocytes, which then produce antiinflammatory cytokines, particularly IL-1Ra, and a milieu of regenerative growth factors as the solution is incubated typically for 6 to 24 hours. The process creates a cell-free solution, autologous conditioned serum (ACS), which is then recovered by centrifugation.
Mechanism of Action and Clinical Applications
The proposed mechanism of action of IRAP is by competitive inhibition at the IL-1 receptor, blocking the pro-inflammatory IL-1 effect. There are no clear indications for the use of autologous IL-1Ra at this time. Some possible indications are in osteoarthritis of major joints. In osteoarthritis specifically, IL-1 directly contributes to cartilage loss through upregulation of extracellular proteolytic enzymes while simultaneously suppressing cartilage anabolism through downregulation of collagen and proteoglycan synthesis. ,
Data on Efficacy
A 2019 systematic review of IL-1Ra products for knee osteoarthritis concluded that autologous IL-1Ra may improve pain and functionality for mild to moderate OA, and may be an effective adjunct for those unresponsive to traditional IA therapies. In a randomized, double-blind, placebo-controlled clinical trial in knee OA patients a one-time injection of IL-1Ra significantly improved pain scores compared to placebo; however, there was no significant difference 4 weeks after the injection. ACS with high-concentration IL-1Ra has also been studied for the treatment of lumbar and cervical radiculopathy with improvement in pain, function, and quality of life similar to that of corticosteroids with epidural injections, but with possibly a longer efficacy. There is early preclinical work in a rat model of tendon disease, where IL1-Ra improved structural endpoints with histologic analysis.
Current research is exploring long-term IL-1Ra delivery by gene therapy, or injecting the gene encoding the protein using a virus vector. This has been proposed to be a superior method of increasing IA IL-1Ra expression. Gene therapy IL-1Ra has been studied in animal models and found to be safe, symptomatically effective, and disease modifying; however, to date, there are no human trials.
FDA Regulations
There is no FDA-approved system to produce autologous whole-blood–derived IL-1Ra proteins at this time. It is the opinion of the author that the Orthokine system (or Regenokine in the United States) to produce ACS is more than minimally manipulating the whole blood product, and thus would not categorize under regulatory exemption per 21 CFR 1271 and the 361-product exemption.
Bone Marrow Aspirate Concentrate
Bone marrow aspirate concentrate (BMAC), also known as bone marrow concentrate (BMC), is a preparation of harvested autologous bone marrow. After harvesting, centrifugation is used to concentrate MSCs, hematopoietic cells (HCSs), platelets, and bioactive molecules such as cytokines. Similar to PRP, BMAC utilizes cytokines to harness the body’s ability for tissue healing. Unlike PRP, BMAC utilizes multipotent stem cells that can theoretically differentiate into damaged cell types, and also indirectly assist with healing by stimulating angiogenesis and recruiting local tissue-specific progenitor cells through paracrine effects. It is important to note that there has been efforts to rename what is currently known as mesenchymal stem cells to medicinal signaling cells. Dr. Caplan, one of the pioneers to first coin the term “mesenchymal stem cells” in the 1970s–80s, has suggested now using the new terminology to more accurately reflect that the cells migrate to the sites of injury or disease and then secrete bioactive factors. It appears that the MSCs do not necessarily function in the body as progenitor cells for new tissues, and instead the bioactive factors secreted by the exogenously supplied MSC stimulate the patient’s own site‐specific and tissue‐specific resident stem cells to construct the new tissue. Currently, there is no clear consensus on the mechanism of action of these cells and how they ultimately influence the observed effects on the tissue microenvironment.
The International Society for Cellular Therapy (ISCT) proposed that MSCs must be plastic-adherent (such as to a tissue culture flask); must express the surface markers CD73, CD90, and CD105 and not express the hematopoietic markers CD14, CD34, CD45, CD11b, CD79a, CD19, or HLA class II; and should be able to undergo multilineage differentiation (osteogenic, adipogenic, and chondrogenic) in vitro. MSC’s low immunoreactivity and high immunosuppressive properties make it a promising stem cell source for therapy.
The two currently available bone marrow stem cell preparations are cultured bone marrow stem cell (referred to in the text as BMSCs) and noncultured BMAC that contains MSCs but also a variety of other cells (referred to in the text as BMACs). Cultured BMSCs require a two-step procedure for expansion of BMSCs in vitro to increase cell counts to 100- to 10,000-fold during several weeks in culture. , In this section, we will be focusing on non-cultured BMACs, with a subsequent section elaborating on cultured BMSCs.
BMAC is harvested from the patient’s bone marrow, most commonly from the posterior iliac crest, due to higher yield, though other sites include tibia, femur, sternum, and humerus. , The physician inserts a needle percutaneously into the posterior iliac crest, past the cortical bone and into the medullary cavity to obtain bone marrow. Detailed techniques, yield, and additional information on bone marrow harvest are described in Chapter 6 of this text.
BMAC is a concentrated milieu of MSCs, HCSs, pericytes, endothelial progenitor cells (EPCs), osteochondroreticular (OCRs) skeletal progenitor cells, stromal cells, multilineage-differentiating stress-enduring (Muse) cells, platelets, and various cytokines such as but not limited to IL-1RA, PDGF, and VEGF. Cell components include erythroblasts, neutrophils, eosinophils, basophils, monocytes, lymphocytes, macrophages, plasma cells, and megakarykocytes. , The end composition is reduced in RBCs and blood plasma. It is important to note that while there are multiple commercial BMAC devices available to the practitioner, the composition and consistency of the preparations vary. , Cassano and colleagues noted a significant difference in the IL-1B, TGF-β, and PDGF concentration between two commercial BMC systems. , Dragoo and Guzman compared three commercially available BMAC preparation systems in a controlled laboratory study with 10 patients. While the authors noted a variation in the WBC concentration consistency between two systems, there was not a significant difference in consistently among the platelet, CD34+ cells concentration, and colony-forming unit (CFU) fibroblasts. However, the composition of the concentrate products did differ across the systems.
Mechanism of Action and Clinical Applications
While the exact mechanism of how BMAC functions is not known, the various components of BMAC have been shown to induce differentiation and proliferation of resident stem cells, and have chondrogenic and osteogenic potential, as well as antiinflammatory effects in vitro. HCSs, also found in BMAC, can differentiate into red blood cells and muscle, are involved in muscle repair, and stimulate osteogenesis with direct contact with MSCs. , In addition to the platelet’s ability to release growth factors to initiate stem cell migration to the injury site and provide adhesion sites for migrating stem cells, platelets can also decrease pain via a peripheral endocannabinoid related pathway. ,
There is evidence that the dose of progenitor cells can affect clinical outcomes. Hernigou reported that for the treatment of nonunion tibia fractures with BMC, the total number and concentration of bone marrow progenitor cells had a significant effect on fracture healing. Pettine reported that in the treatment of discogenic pain with BMC, higher MSC concentration was linked to pain reduction post procedure. Centeno reported that in the treatment of knee arthritis with BMC, in patients with a total nucleated cell (TNC) count above 400 million there was significant improvement in all studied pain and functional metrics compared to the lower cell count group. Both groups did report significant improvement from baseline.
The addition of a supplementary PRP injection to the BMAC treatment protocol can potentially influence the results of an isolated BMAC injection for OA. A single, image-guided IA injection of BMAC followed by a supplementary IA injection with PRP at 8 weeks follow-up was well-tolerated and significantly improved pain at short-term follow-up among patients with moderate-to-severe OA. An ongoing clinical trial called the CASCADE trial is examining the use of BMAC with and without HA for discogenic low back pain. ,
Data on Efficacy
Few studies have compared the use of noncultured (nonexpanded) BMSCs with cultured (expanded) stem cell therapy, and it is unclear which method may be superior. While there are no RCTs on the efficacy of noncultured BMAC for patients with cartilage disease, there have been many publications illustrating its safety profile and therapeutic potential. Furthermore, the harvested stem cell quantity and quality can vary by patient, which makes research comparisons difficult. Baseline pain and functional limitations, BMAC dose, PRP dose (if given at the same time), and individual biologic factors can all influence treatment efficacy.
Some of the current indications and uses for BMAC under evaluation include chondral defects of the knee, full-thickness and moderate-to-large cartilage defects of the knee, osteoarthritis of the knee, meniscal tears, spine-mediated pain, partial tear of the rotator cuff tendon, , shoulder arthritis, osteochondral lesions of the talus, ACL repair, hip arthritis, and hip osteonecrosis. Autologous BMAC has also been used intraoperatively as an adjunct to debridement or microfracture surgery for cartilage defect and has been shown to improve healing and reduce rotator cuff retears when augmenting RTC surgery.
Studies of autologous BMC for the treatment of IA knee pathology are among the most numerous. Shapiro and colleagues performed a prospective, single-blind, placebo-controlled trial that compared BMAC and saline placebo injection for patients with bilateral knee osteoarthritis and found no serious adverse events from the BMAC procedures as well as a significant decrease in VAS pain scores at 1 week, 3 months, and 6 months.
With respect to the spine, there is an increasing number of studies. Pettine and colleagues examined outcomes in 26 patients following intradiscal percutaneous injection of BMC for discogenic low back pain. At 3-year follow-up, six patients went on to surgery. Of the remaining 20 patients, average ODI improved from baseline of 56.7 to 17.5 and average VAS improved from 82 to 22 at 36 months. At 1 year, 40% of patients had an improvement of one modified Pfirrmann grade on magnetic resonance imaging (MRI) and no patients had worsening of their imaging.
Caveats/Side Effects
Adverse reactions include general procedural risks, including but not limited to pain, swelling, bleeding, infection, damage to nearby structures, and potential detrimental effects of erythrocytes when used for IA joints. Contraindications include bone marrow–derived cancer (lymphoma), active systemic infection, blood thinners, and non-bone marrow–derived cancers which is a relative contraindication. However, a study by Hernigou and colleagues found no increased cancer risk in patients after application of autologous cell-based therapy using bone marrow–derived stromal progenitor cells either at the treatment site or elsewhere in the patients after an average follow-up period of 12.5 years.
Centeno and colleagues performed a large prospective study on 339 patients who were treated for various orthopedic conditions with culture-expanded autologous, bone marrow–derived MSCs that were harvested from the posterior superior iliac crest between 2006 and 2010. The mean patient age was 53 ± 13.85 years (214 males and 125 females) and follow up varied from 3 to 36 months. Using high-field MRI tracking and complications surveillance, they noted that while there were two patients who reported the development of cancer, the cancers were not at the injection sites and the percentage of patients that developed cancer was not far from the annual rate of cancer in the general population. The most common complaints were pain and swelling (2% of patients), with no reports of infections and procedure-related complications were self-limited or remediated with simple therapeutic measures. Centeno and colleagues subsequently performed a larger prospective multicenter study investigating the safety of same day autologous BMC aspiration isolation, and injection (n = 1590 patients, 1949 injections), same day autologous BMC aspiration, isolation and injection with an addition of minimally processed lipoaspirate (n = 247 patients, 364 injections), and cultured expanded BMSCs re-implanted weeks or months after bone marrow aspiration (n = 535 patients, 699 injections), orthopedics procedures across 18 sites. The discrepancy in the number of procedures and patients can be explained by multiple joint procedures that occurred during the same session, and/or serial injections occurring at different treatment sessions for the same patient. 3012 procedures were performed over 9 years with 2372 patients reporting on adverse advents (AEs) with a mean follow up time of 2.2 years. Follow-up ranged from 1 month to 8.8 years with an average of 2.2 years. 107 A total of 325 (12.1%) AEs were reported, with 38 adjudicated to be definitely related to the procedure (1.6% of the population). The majority of the AEs were post-procedure pain or pain due to degenerative joint disease. Thirty-six (1.5% of the population) reported a serious side effect, with 19 of those adjudicated to be not related to the procedure and 4 definitely related. There were 7 neoplasms reported with none at the site of injections. The BMC only group was less likely to report AEs compared to the BMC with addition of minimally processed lipoaspirate and culture BMSC groups.
Blood-thinning medications such as coumadin and aspirin may be discontinued and managed appropriately by the cardiologist or primary doctor prior to the procedure. Lastly, the financial costs associated with BMAC procedures may be prohibitive for some patients as they are often not covered by insurance for elective orthopedic procedures.
FDA Regulations
There are two currently available BMSC preparations: cultured BMSCs and noncultured (nonexpanded) BMACs. The culturing method is currently prohibited in the United States as current regulatory guidelines consider in vitro expansion of the BMA more than “minimally manipulated.” In contrast, noncultured BMACs do not require laboratory cell expansion and culturing, and are available after centrifugation for same-day treatment. Given that this is a single-step process with “minimal manipulation” of cells, this meets the criteria established by the US FDA for homologous use. , As defined in 21 CFR 1271.3(c), “homologous use means the repair, reconstruction, replacement, or supplementation of a recipient’s cells or tissues with an HCT/P that performs the same basic function or functions in the recipient as in the donor.” Bone marrow meets this criterion for orthopedic uses as its constituents are involved in bone, cartilage, tendon, and muscle repair. Care should be taken when processing BMAC to not combine the BMAC with other agents, except for water, crystalloids, or a sterilizing, preserving, or storage agent to satisfy the minimally manipulated guidelines.
Minimally manipulated BMAC used for cartilage resurfacing therapies is not regulated by 21 CFR 1271 and therefore does not require premarket approval, preclinical research, or clinical trials to be marketed for treatment in the United States. , The FDA released a guidance document in November 2017 to explain the regulatory demands of manufacturers of human cells, tissue, and cellular and tissue-based products by specifically delineating the definition of “minimal manipulation.” , BMAC preparation kits and concentration systems require only a 510(k) Premarket Notification to the FDA 90 days before marketing the product. Thus, it highlights the importance of the clinician to counsel the patients effectively on the risks, benefits, and potential outcomes.
Adipose Tissue
Since the first isolation and classification of adipose tissue stem cells (ASCs), human studies related to ASCs have increased, reaching up to 187 clinical trials in 2015 and more registered trials in 2019. Subcutaneous adipose tissue is a common reservoir of progenitor cells, such as MSCs, and the clinical application of fat has been used in cosmetic, reconstructive, and corrective indications. MSCs have vast potential in regenerative medicine due to their ability to proliferate and differentiate into multiple lineages, including adipocytes, chondrocytes, myocytes, and osteoblasts. , MSCs can be obtained from different sources, including bone marrow, adipose tissue, dental pulp, synovium, muscle, and other tissues. The evidence and proliferation of human use have vastly expanded since the first isolation and description of the progenitor cells in rodents in 1964 by Rodbell.
Current applications have included knee osteoarthritis, osteonecrosis of the femoral head, hip osteoarthritis, and shoulder osteoarthritis and rotator cuff pathologies. Some studies have assessed ASCs in varying conditions in human and animal models, such as multiple system atrophy, Crohn disease, cardiac disease, ischemic heart disease, amyotrophic lateral sclerosis, Parkinson’s disease, , spinal cord injury, rheumatoid arthritis, type 1 diabetes mellitus, type 2 diabetes mellitus, and Alzheimer disease. ,
Methods for harvesting adipose tissue include resection, tumescent, conventional liposuction, and ultrasound-assisted liposuction (UAL; these are covered in more details in Chapter 5 : Autologous Tissue Harvesting Techniques: Bone Marrow Aspirate and Adipose Tissue). , After harvesting, the raw lipoaspirate can be processed using various methods and protocols to produce an injectable MFAT, enzyme digested stromal vascular fraction (SVF), or cultured ASC product. , When the adipose tissues are further processed into the SVF or cultured ASC, they are considered more than minimally manipulated and do not meet the current regulations and criteria established by the FDA. Information regarding SVF and ASCs will be described in further detail in the subsequent section under cultured cellular injectates. There are FDA-compliant devices that can make MFAT with intact stromal vascular niche and MSCs that can then be injected back into the patients, such as Lipogems, PureGraft, and IntelliFat.
MFAT preserves the stromal vascular architecture and stem cell niche; however, robust studies that have assessed its molecular profile are lacking. SVF has been better characterized, and is composed of a heterogeneous mixture of cells including pre-adipocytes, pericytes, mast cells, fibroblasts, smooth muscle cells, endothelial cells, hematopoietic stem cells, and adipose tissue–derived mesenchymal stem cells (AT-MSCs). , Adipose tissue can yield up to 500,000 to 2,000,000 cells per gram of tissue.
The AT-MSC yield, proliferation, and differentiation capacity can be influenced by the location of adipose tissue harvested, the method of harvesting, the age of the patient, and other factors such as donor’s body mass index. , Adipose has a high heterogeneity among individuals and within the same individuals with the various stem/precursor cells, different types of adipocytes (white, beige, brown), endothelial cells, and even pericytes. The quality of AT-MSCs can also fluctuate based on the purifying method and storage conditions.
The most common locations to extract adipose tissue include the abdomen and hip/thigh region, with the infrapatellar fat pad being a less commonly used location. , , More studies illustrate that the abdomen is the optimal location to harvest adipose tissue. ,
Depending on the methods of extracting adipose tissue, the yield, number of viable cells, and growth characteristics of AT-MSCs may differ. The viability of ADSCs may be affected by the stress applied to the adipose tissue during harvesting and processing. Oedayrajsingh-Varma and colleagues compared adipose tissue resection, tumescent, conventional liposuction, and UAL, and their effects on the ACS yields and growth characteristics. The number of viable cells in SVF was similar regardless of the harvesting technique, but UAL contained fewer stem cells, and viable stem cells had a longer population doubling time. Keck and colleagues compared power-assisted liposuction with manual aspiration in nine subjects undergoing abdominoplasty. Both conditions had a comparable number of viable ASCs per mL of aspirated fat, similar proliferation rates, and ability to differentiate into mature adipocytes, though the cells harvested using power-assisted liposuction had significantly higher expression levels of differentiation markers adiponectin, GLUT4, and PPARg. Duscher and colleagues compared an UAL device versus standard suction-assisted lipoaspiration in three patients with paired collection. They reported that both methods had comparable ASC yield, viability, osteogenic and adipogenic differentiation capacity, and certain cytokine expressions such as VEGF, hepatocyte growth factor, stromal cell–derived factor 1, and bFGF, though the UAL method had more expression of the monocyte chemotactic protein 1.
The effectiveness of AT-MSCs when obtained from and utilized in elderly patients must be considered. Choudhery and colleagues noted that aged AT-MSCs displayed senescent features when compared with cells isolated from young donors, and also exhibited reduced viability and proliferation. The authors noticed a significant drop in the osteogenic potential of AT-MSCs with increased donor age; however, the adipogenic and the neurogenic potential of the AT-MSCs seemed to be maintained during aging. Schipper and colleagues assessed the composition of cells harvested from 12 female patients within 3 age ranges (25 to 30, 40 to 45, and 55 to 60 years old) and from 5 different subcutaneous adipose depots. They observed sensitivity to apoptosis was linked to the anatomic depot, with the superficial abdominal depot (above Scarpa layer) more resistant to apoptosis compared to the other sites (deep abdominal layer, arm, thigh, and trochanteric). They also noted faster cell proliferation rates in younger patients than the older age groups, and that younger patients had increased peroxisome proliferator activated receptor (PPAR)-γ-2 expression, which is required for adipogenesis both in vivo and in vitro. The older patients only have elevated PPAR-γ-2 expression in the arm and thigh depots.
In addition to age and harvest location influencing the composition and effectiveness of AT-MSCs, a systematic review by Varghese and colleagues noted decreased proliferation and differentiation potential of the AT-MSCs with increasing body mass index, diabetes mellitus, exposure to radiotherapy, and tamoxifen. In one study, ASCs in adipose tissue of obese patients had lower capacity for repair than ASCs from nonobese patients, likely due to higher incidence of metabolic syndrome in obese patients. Another study showed that ASCs from obese donors had shorter life spans, increased DNA damage, and more adipogenic differential potential than ASCs from normal-weight donors. ASCs from type 2 diabetics showed higher levels of senescence and apoptosis, and reduced osteogenic and chondrogenic potential, while enhancing adipogenic potential.
Furthermore, it appeared that there is not a significant impact of hypertension, renal disease, physical exercise, peripheral vascular disease, and total cholesterol on AT-MSC yield, though the authors noted it is very difficult to make conclusive recommendations due to the variability in studies.
Mechanism of Action and Clinical Applications
MSCs are adult stem cells that can differentiate into a variety of cell types, including osteoblasts, chondrocytes, and adipocytes. , MSCs assist with tissue regeneration by differentiating into damaged cell types or indirectly by stimulating angiogenesis, limiting inflammation, and recruiting local tissue-specific progenitor cells. MSCs also secrete growth factors and cytokines, including but not limited to prostaglandins, TGF-B1, nitrous oxide, IL-4, IL-6, and IL-10, and IL-1 receptor antagonist. , HCSs can support the vascular system by differentiating into blood cells and stimulating osteogenesis with direct contact with MSCs. A study by Ceserani and colleagues on MFAT harvested using Lipogems Systems reported that the MFAT has the capacity to induce vascular stabilization and even retain antiinflammatory effects.
A study by Vezzani and colleagues noticed that the microanatomy of MFAT is similar to that of intact adipose tissue, with capillaries, microvessels, and pericytes wrapped around endothelial cells; however, they noted a higher frequency of pericytes in MFAT than in manual lipoaspirate and SVF. It is notable that recent literature has suggested that in vivo the main functionality of MSCs may not be multipotency. While pericytes have been widely believed to function as MSCs, others speculate that all MSCs could be pericytes due to the locations and cell marker signatures. Crisan and colleagues observed the expression of MSC markers on the surface of perivascular cells, suggesting blood vessel walls may harbor a reserve of progenitor cells. Guimarães-Camboa and colleagues’ lineage-tracing experiments suggest that the plasticity observed in vitro, or following transplantation in vivo, may be due to the artificial cell culture environment.
Microvascular fragments from adipose tissue exhibit a high angiogenic activity, representing a rich source of MSCs, and can rapidly develop into microvascular networks. When the resulting MFAT is processed, such as with Lipogems, pericytes can be retained within the intact stromal vascular niche as well as retaining the MSCs.
AT-MSCs isolated from younger donors are anticipated to be a more useful cell source for tissue engineering and regenerative medicine applications. There is theoretical potential for cell-based therapies for the elderly by banking adipose tissue at a younger age, preserving the stem and progenitor cells when biologic activity is at its greatest potential.
Acknowledging a need for further high-quality research, the American Academy of Orthopaedic Surgeons in 2018 collaborated and called for establishing high-quality patient registries that can assist with continued surveillance and quality assessment.
Caveats/Side Effects
Adverse reactions include general procedural risks including but not limited to pain, swelling, bleeding, infection, and damage to nearby structures. Kuah and colleagues evaluated the safety and tolerability of in vitro expanded MSCs derived from human donor adipose tissue combined with cell culture supernatant, and did not find any severe side effects when administered as a single IA injection to patients with symptomatic knee osteoarthritis. Swelling of injected joints can be a common side effect, and is thought to be associated with the death of stem cells. Other potential side effects, though less common, include tenosynovitis and tendonitis, arthralgia, joint stiffness, effusion, and paresthesia or malaise. , ,
Theoretically, MSCs can divide into unwanted oncologic cell lineages, and could contribute to tumor behavior by influencing the tumor microenvironment and promoting angiogenesis; however, this has not been seen with adult-derived MSCs. No literature documenting neoplastic complications at ADSC implantation sites have been reported, and a longitudinal cohort study by Pak and colleagues on SVF with PRP into various joints found no evidence of neoplastic complications in any implantation site.
The cost of the procedure is generally not covered by insurance, and can be prohibitive for some.
FDA Regulations
There are some FDA-compliant devices that can make MFAT with intact stromal vascular niche and MSCs such as Lipogems, PureGraft, and IntelliFat, which can then be injected back into the patients through the use in orthopedic applications, and whether it counts as homologous use is debatable. The FDA defines the basic function of adipose tissue as providing cushioning and support. Some argue MFAT acts to cushion and/or support orthopedic tissue injuries, such as tendon and meniscal tears, and should be considered homologous.
Recently published guidelines that indicate enzymatically or mechanically processed lipoaspirate (PLA) alters these relevant characteristics and is not considered homologous use or minimal manipulation by the FDA. , SVF is adipose tissue that undergoes further processing (such as with enzymatic digestion) to break down and eliminate the adipocytes and surrounding structural components that provide cushioning and support, and thus is considered more than minimally manipulated. , Further laboratory cell sorting and culture expansion would also be considered more than minimally manipulated due to the steps involved in isolating and culturing of cells. Adipose tissue that is processed into SVF or cultured ASC is more than minimally manipulated and not FDA approved based on the current guidelines. Minimally manipulated ADSC used for cartilage resurfacing therapies is not regulated by 21 CFR 1271 and therefore does not require premarket approval, preclinical research, or clinical trials to be marketed for treatment in the United States. Consequently, some products are not FDA approved and may qualify instead for investigational new drug process.
Bone Marrow–Derived Versus Adipose-Derived Stem Cells
MSCs can be obtained from different sources, including bone marrow, adipose tissue, dental pulp, synovium, muscle, and other tissues. To date, bone marrow–derived MSCs are the best characterized and studied. Recently, other MSC sources, particularly adipose tissue, have gained clinical interest for use in regenerative medicine. , Zuk and colleagues were one of the initial researchers to assess PLA cells obtained from liposuctioned adipose tissue, and found it represented a potential source of multilineage mesodermal stem cells. Few studies have compared the efficacy of BMAC and MFAT. ,
There are vast differences in the reported stem cell yields in adipose and bone marrow, with some studies reporting 100 to 500 times higher stem cell numbers found in adipose versus bone marrow. However, based on donor-matched studies the differences volume per volume or gram for gram appear far less than that. In one study, patients undergoing ACL reconstruction donated samples of several tissues including bone marrow and adipose. Bone marrow had about 93× more TNCs per volume compared to adipose (2045 vs 22). Adipose had about 148.5× more CFUs per 1000 TNCs, but bone marrow had the most cells per colony. Given that bone marrow had more TNCs, adipose contained about 1.6× as many MSCs per volume compared to bone marrow. BMC was also found to contain 6x more nucleated cells compared to SVF. SVF contained 4× more adherent cells. Colony-forming frequency was 0.5% in SVF versus 0.01% in BMC. MSC population was 4.28% of the TNC in SVF versus 0.42% in BMC or about 10× more in SVF. In summary, bone marrow has more nucleated cells than adipose but only a small percentage are MSCs. Adipose has less nucleated cells but a higher percentage of those are MSCs. Pending variability in harvesting techniques and patient characteristics, adipose per volume likely has 1.6 to 10× as many MSCs as bone marrow. It has been reported that the lipoaspirate harvesting, when compared to bone marrow harvesting, can yield more absolute MSCs, the MSCs have faster rapid in vitro expansion, and may yield more stem cells per gram of tissue. , ,
While there are similar and varying cell surface marker profiles between bone marrow–derived mesenchymal stem cells (BM-MSCs) and adipose tissue–derived mesenchymal stem cells (AT-MSCs), they both have the potential to differentiate into multiple lineages, including osteogenic, chondrogenic, adipogenic, cardiomyocytic, hepatic, and neurogenic differentiation. , ,
BM-MSCs and AT-MSCs have comparable immunophenotypes; however, AT-MSCs retain their phenotype more consistently over BM-MSCs across multiple culture passages, and also have higher proliferation capacities in part due to their lower senescence ratio. A donor-matched study comparing culture-expanded AT-MSCs vs BT-MSCs showed that AT-MSCs underwent faster proliferation and doubling times. They had longer life spans possibly due to the fact they had longer telomeres, which may suggest a smaller dose is required in vivo. Both had similar differentiation capacity and decreased proliferation the longer the in vitro expansion was performed. On the other hand, Mohamed-Ahmed and colleagues found BM-MSCs were superior to AT-MSCs in terms of osteogenic and chondrogenic differentiation, while AT-MSCs had higher proliferation and adipogenic potential. Chondrogenesis was more efficient in human BM-MSCs versus AT-MSCs in vitro. Human BM-MSCs showed more chondrogenic potential in vitro compared to AT-MSCs. Xu and colleagues noted that BM-MSCs possessed stronger osteogenic and lower adipogenic differentiation potentials compared to AT-MSCs, but found no significant difference in the chondrogenic differentiation potential. Xu and colleagues’ results demonstrated that the DNA methylation status of the main transcription factors controlling MSC fate influenced their expression, and subsequently different differentiation capacities of AT-MSCs and BM-MSCs.
Clinically, there are limited clinical data comparing BMAC to MFAT. Mautner and colleagues conducted a retrospective study of 110 patients comparing the pain and functional outcomes of patients with symptomatic knee osteoarthritis who received BMAC or MFAT or injections. At a mean follow-up time for BMAC of 1.8 years and 1.0 years for MFAT, they noted both groups had significant improvement in Emory Quality of Life, VAS for pain, and Knee Injury and Osteoarthritis Outcome Score questionnaire ( P < .001) without a significant difference between BMAC and MFAT.
Cultured Cellular Injectates
Mesenchymal stem cells/stromal cells (MSCs) have a vast potential in regenerative medicine due to their ability to proliferate and differentiate into multiple lineages including adipocytes, chondrocytes, myocytes, and osteoblasts. Cultured cells are in theory attractive, given their ability to potentially differentiate to the desired cell type of an injured tissue. MSCs can be obtained from different sources.
Mesenchymal Stem Cells/Stromal Cells From Bone Marrow
After harvesting BMA, the sample is processed in a centrifuge and subsequent buffy coat layer and platelet-poor plasma layers are removed. The cells are subsequently isolated, generally by their adherence to plastic, and then cultured. , Culturing methods can vary, though one method by DiGirolamo and colleagues includes diluting the aspirate marrow with Hank’s balanced salt (HBS; Gibco), washing gently with inversion, adding a layer of Ficoll (Ficoll‐Paque; Pharmacia) beneath the sample, and then spinning the sample at 2500 g for 30 minutes at room temperature. The mononuclear cell layer was then washed again with HBS and the cells spun at 1500 g for 15 minutes and resuspended in complete medium (Minimum Essential Medium, alpha medium without deoxyribonucleotides or ribonucleotides, Gibco; 20% fetal calf serum lot‐selected for rapid growth of human marrow stromal cells (hMSCs) FCS, Atlanta Biologicals; 100 units/mL penicillin, 100 μg/mL streptomycin, Gibco; and 2 mm L‐glutamine, Gibco). Cells were then plated in a 25 cm 2 tissue cultured flask (Nunc), incubated at 37°C with 5% humidified CO 2 for 1 day before the non-adherent cells were removed. Adherent cells were then washed twice with PBS and shaken to remove adherent hematopoietic precursors, and fresh complete medium was added. The medium was replaced every 3 to 4 days until the cells grew to 70% to 90% confluency, and harvested with trypsin and ethylenediaminetetraacetic acid (EDTA), with the entire process generally repeated to expand the cells through various passages.
Mesenchymal Stem Cells/Stromal Cells From Adipose Tissue
After harvesting adipose tissue, the raw lipoaspirate can be processed using various methods and protocols to produce an injectable, including MFAT, enzyme-digested SVF, or cultured ASC. , After isolation from the raw lipoaspirate (refer to formulation section in Adipose Tissue section above), the SVF can either be used directly in clinical procedures or can be cultured to increase the number of cells before using them clinically. With cell culturing, only ASCs and their precursor cells (supra-adventitial cells and pericytes) are able to adhere and survive. , It is thought that the predominant benefit of SVF relies on the presence of ASCs. The process of isolating and culturing ASCs is generally a long procedure that is not performed at the same time as surgery. ,
When the adipose tissues are further processed into the SVF or cultured ASC, they are considered more than minimally manipulated and do not meet the criteria established by the FDA and do not comply with current regulations. To make SVF, the raw lipoaspirate is washed with PBS and subsequently undergoes enzymatic digestion with collagenase, dispase, trypsin, or other enzymes (ranging from 30 minutes to 1 to 2 hours). After neutralization of the enzymes, the remaining elements are now called the stromal vascular fraction or SVF, and are separated from the mature adipocytes by centrifugation. The SVF is composed of a heterogeneous mixture of cells, including pre-adipocytes, pericytes, mast cells, fibroblasts, smooth muscle cells, endothelial cells, hematopoietic stem cells, and AT-MSCs. ,
When SVF cells are cultured, a subset of cells adheres to the tissue culture plasticware. After purification with washing and culture expansion with media, the HCSs (nonplastic adhesive cells) are separated from the SVF cells, leaving behind the adipose tissue–derived stromal cells which include the stem cells, which can be expanded in a culture medium. , The cells are then resuspended in a mixture of culture medium and cryoprotective medium and frozen and stored under good manufacturing practice. Cells can then be expanded in a culture medium for extended periods and utilized later. , Digesting the triple helix region of the peptide bonds in the collagen of adipose tissue with collagenase can be both time consuming and expensive. In some cases, it may be possible to obtain ASCs without enzymatic digestion. , Baptista and colleagues isolated a population of MSC from lipoaspirate samples without tissue digestion and found that it is possible to store freshly isolated mechanically processed lipoaspirate adipose tissue (MPLA) cells by cryopreservation without a significant loss of MSCs.
Jurgens and colleagues investigated whether the yield and functional characteristics of ASCs are affected by the adipose tissue-harvesting site and reported that the percentage of ASCs in the SVF of adipose tissue harvested from the abdomen was significantly greater than those harvested from the hip/thigh region ( P < .05). The authors noted that while there not was a significant difference in the ASC proliferation or differentiation capacity from cells harvested in the abdomen compared to cells harvested from the hip and thigh, there was a significantly increased yield of ASCs found in SVF of abdominal adipose tissue compared to SVF of adipose from hip/thigh regions. Furthermore, Iyyanki and colleagues similarly found higher total SVF, but not tissue–derived stem cell yields in fat harvested from the abdomen compared to the flank or axilla. Tsekouras and colleagues assessed 53 lipoaspirates in various locations (inner thigh, outer thigh, abdomen, waist, and inner knee) and found that the outer thigh exhibited significantly higher SVF cell count compared to other donor sites and both the inner and outer thigh showed a significantly higher number of ASCs. No significant differences in the viability of SVF cells and ASCs were noted. Fraser and colleagues evaluated the stem and progenitor cell content of subcutaneous adipose tissue in the hips and the abdomen of 10 subjects undergoing elective liposuction. Using clonogenic culture assays and a paired analysis of tissue obtained from the different subcutaneous sites, the authors noted that tissue harvested from the hip yielded a statistically significant 2.3-fold more fibroblast CFU volume and a 7-fold higher frequency of alkaline phosphatase-positive colony-forming unit (CFU-AP, a widely used marker for osteogenesis) than that obtained from the abdomen. One caveat is that the results could reflect the differences in ratio of deep and superficial subcutaneous adipose tissue collected from the two sites, as deep subcutaneous adipose tissue generally has a higher frequency of blood vessels, and thus more pericytes which may be detected by the CFU-AP assay Pericytes, as mentioned before, have been shown to possess an adipogenic potential.
FDA Regulations
Cultured MSCs are currently prohibited in the United States, as the methods for culture and isolation are currently considered more than minimal manipulation of the cells. The culturing method is performed outside of the United States; however, some institutions through special approval for research have been able to utilize culture-expanded cells. The FDA released a guidance document in November 2017 to explain the regulatory demands of manufacturers of human cells, tissue, and cellular and tissue-based products by specifically delineating the definition of “minimal manipulation.”
Conclusion
Culture-expanded MSCs certainly have potential in clinical applications; however, more robust science is necessary to better understand their safety profile and efficacy in musculoskeletal applications.
References
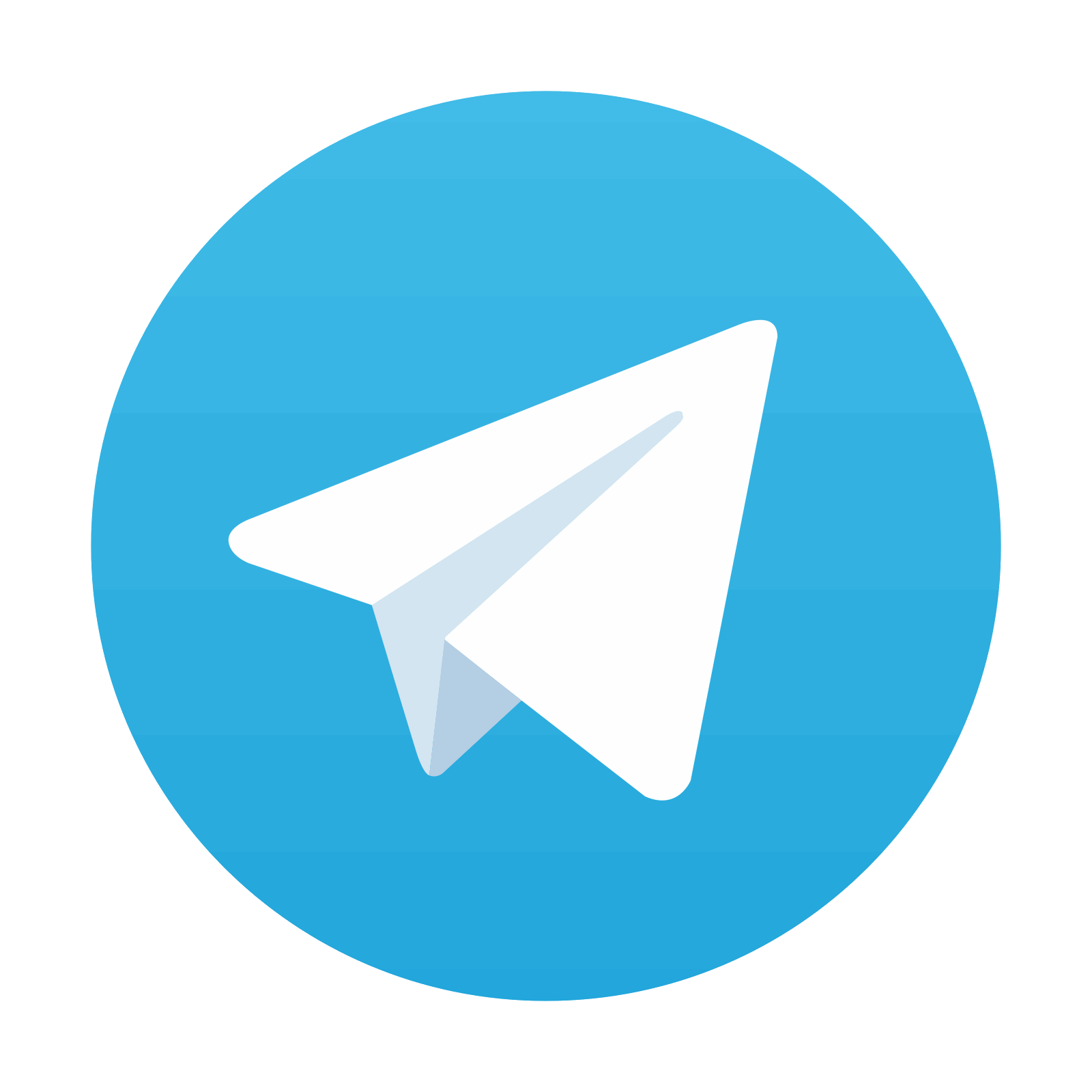
Stay updated, free articles. Join our Telegram channel

Full access? Get Clinical Tree
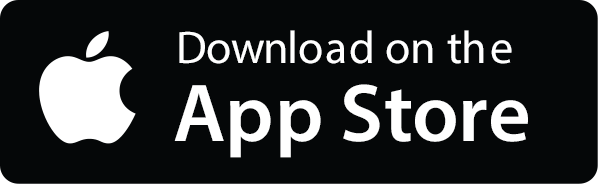
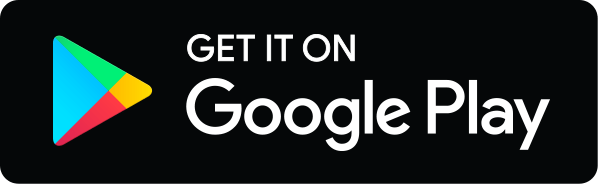
