Arthrofibrosis after periarticular fractures can create clinically significant impairments in both the upper and lower extremities. The shoulder, elbow, and knee are particularly susceptible to the condition. Many risk factors for the development of arthrofibrosis cannot be controlled by the patient or surgeon. Early postoperative motion should be promoted whenever possible. Manipulations under anesthesia are effective for a period of time in certain fracture patterns, and open or arthroscopic surgical debridements should be reserved for the patient for whom nonoperative modalities fail and who has a clinically significant deficit.
Key points
- •
Arthrofibrosis after periarticular fractures can create clinically significant impairments in both the upper and lower extremities. The shoulder, elbow, and knee are particularly susceptible to the condition.
- •
Many risk factors for the development of arthrofibrosis cannot be controlled by the patient or surgeon. Early postoperative motion should be promoted whenever possible.
- •
Manipulations under anesthesia are effective for a period of time in certain fracture patterns, and open or arthroscopic surgical debridements should be reserved for the patient for whom nonoperative modalities fail and who has a clinically significant deficit.
Arthrofibrosis occurs when generalized connective tissue proliferation results in painful joint stiffness. The clinical impact of arthrofibrosis represents a wide spectrum of disease, ranging from minimal to devastating for the patient. Arthrofibrosis may result in a loss of motion of the involved joint, a commonly encountered and challenging problem after fixation of periarticular fractures. Joints across the body tolerate stiffness to a variable degree, with the elbow and knee being less accommodating than the shoulder or ankle. The functional range of motion has been described previously for the shoulder, elbow, and knee, and when arthrofibrosis results in the loss of this functional range, intervention may be indicated. Once arthrofibrosis has progressed, activities of daily living (ADLs) can become compromised, with potential resultant limitations in feeding, hygiene, sitting, and ambulation, depending on the degree of motion loss and the joint involved.
Anatomic articular reduction is critical to minimizing posttraumatic arthritis in many periarticular fractures. Subsequently, many historic rehabilitation protocols called for prolonged periods of immobilization to minimize the risk of fracture displacement during the period of fracture healing. Early motion of the injured joint is now frequently emphasized over a prolonged period of immobilization, as immobilization is a well-established risk factor for the development arthrofibrosis after fixation of periarticular fractures.
The clinical appearance of arthrofibrosis is loss of motion in the flexion or extension or both, and develops as the result of diffuse scar tissue and adhesion formation within a joint. Features specific to the patient, the injury, the surgery, and the postoperative rehabilitation seem to play a role in the development of arthrofibrosis.
Regardless of the joint involved, there are a variety of common risk factors for the development of stiffness after intra-articular fracture fixation. Examples include high-energy fracture patterns, the use of an external fixator, prolonged immobilization, and delayed or poor rehabilitation. The development of an exaggerated pathologic fibrous hyperplasia, however, is not always associated with an overt, identifiable cause. Basic science research has shown that certain cytokines, inflammatory cells, and genetic predisposition play a role in the development of postoperative motion loss.
In this article, we discuss the basic science behind arthrofibrosis, review different anatomic areas while highlighting fractures prone to developing postoperative stiffness, and consider the available treatment options.
Basic science
Scar tissue formation occurs as a normal physiologic response to injury and surgical trauma. Tissue organization after surgery depends on the interplay between local and inflammatory cells, with signaling of growth factors and cytokines playing an integral role. The development of arthrofibrosis is in part the result of excess deposition of matrix proteins such as collagen types I, III, V, and the proliferation of fibroblasts. Specific cytokines that play a key role in the development of excessive fibrous hyperplasia and resultant stiffness have been identified. Transforming growth factor-β (TGF-β) and platelet-derived growth factor (PDGF) are 2 examples of signaling molecules that play a critical role in the process of tissue repair. These factors coordinate cell growth, differentiation, and programmed cell death via paracrine and autocrine signaling pathways. At the site of injury, TGF-β and PDGF initiate a cascade of events resulting in the production of extracellular matrix proteins and protease inhibitors, as well as inhibition of proteolytic enzyme production. Formation of extracellular matrix occurs at the site of injury, consisting of an aggregation of collagen, fibronectin, and proteoglycans. With an increase in local concentration, the autoregulatory mechanism of TGF-β results in feedback inhibition. Overexpression of TGF-β can result in progressive deposition of matrix and tissue fibrosis.
In addition, genetic factors likely also contribute to the development of arthrofibrosis. Studies have shown a possible correlation between specific HLA types and the development of arthrofibrosis after anterior cruciate ligament reconstruction. Further investigation is required to confirm a possible genetic link.
Basic science
Scar tissue formation occurs as a normal physiologic response to injury and surgical trauma. Tissue organization after surgery depends on the interplay between local and inflammatory cells, with signaling of growth factors and cytokines playing an integral role. The development of arthrofibrosis is in part the result of excess deposition of matrix proteins such as collagen types I, III, V, and the proliferation of fibroblasts. Specific cytokines that play a key role in the development of excessive fibrous hyperplasia and resultant stiffness have been identified. Transforming growth factor-β (TGF-β) and platelet-derived growth factor (PDGF) are 2 examples of signaling molecules that play a critical role in the process of tissue repair. These factors coordinate cell growth, differentiation, and programmed cell death via paracrine and autocrine signaling pathways. At the site of injury, TGF-β and PDGF initiate a cascade of events resulting in the production of extracellular matrix proteins and protease inhibitors, as well as inhibition of proteolytic enzyme production. Formation of extracellular matrix occurs at the site of injury, consisting of an aggregation of collagen, fibronectin, and proteoglycans. With an increase in local concentration, the autoregulatory mechanism of TGF-β results in feedback inhibition. Overexpression of TGF-β can result in progressive deposition of matrix and tissue fibrosis.
In addition, genetic factors likely also contribute to the development of arthrofibrosis. Studies have shown a possible correlation between specific HLA types and the development of arthrofibrosis after anterior cruciate ligament reconstruction. Further investigation is required to confirm a possible genetic link.
Arthrofibrosis around the shoulder
Functional range of motion of the shoulder requires approximately 120° of forward elevation, 45° of extension, 130° of abduction, 115° of cross-body adduction, 60° of external rotation, and 100° of internal rotation. This motion is significantly less than what has been described as normal shoulder motion (forward elevation 167°, extension 62°, abduction 184°, adduction 140°, external rotation 104°).
Proximal Humerus Fractures
Although some degree of motion loss can be expected after fixation of proximal humerus fractures, with normal motion of the wrist and elbow, the upper limb is typically able to easily accommodate for limited motion without significant functional impairment. Südkamp and colleagues reported the results of open reduction and internal fixation (ORIF) with a locked plate in a prospective, multicenter, observational study. At 1 year follow-up, mean shoulder motion demonstrated 132° forward elevation, 122° abduction, 45° external rotation, and 77° internal rotation. When compared with the uninjured shoulder at 1 year, the shoulder that underwent internal fixation of the proximal humerus had 81% of forward elevation, 79% of abduction, 74% of external rotation, and 93% of internal rotation. These results were consistent with another series that used locked plating of the proximal humerus with the addition of suture fixation, which reported an average forward flexion of 131° in 81 patients at 23 months average follow-up. Duralde and Leddy published their results after plate fixation of proximal humerus fractures also using suture fixation to augment the repair and had an average forward elevation of 156° and 46° of external rotation at an average of 37 months.
A systematic review commented that most of the postoperative rehabilitation protocols of the series of locked plate fixation of proximal humerus fractures were similar, using passive motion initially and active motion 4 weeks later, depending on bone quality and the stability of the fixation.
Postoperative range of motion focusing on daily terminal stretching programs may require several months before maximum benefit is seen. Strengthening of the shoulder should be performed once the clinical and radiographic findings are consistent with fracture healing. Forced manipulation carries the risk of refracture and is rarely required around the shoulder after fixation of proximal humerus fractures. Rarely, postoperative motion loss progresses to adhesive capsulitis that is refractory to conservative treatment. In these instances, shoulder arthroscopy with capsular release is a useful tool that has been shown to improve shoulder motion while allowing the surgeon to evaluate and treat intra-articular conditions. Katthagen and colleagues reported on 37 patients with unsatisfactory results after locked plate fixation of proximal humerus fracture who underwent arthroscopic implant removal with the addition of capsular release (31/37) as necessary. The range of motion improved from 86° to 105° in forward flexion, 77° to 97° in abduction, 30° to 42° in external rotation, and 58° to 81° in internal rotation at 24 months from arthroscopy.
Arthrofibrosis around the elbow
Joint stiffness is a common occurrence after fixation of periarticular fractures involving the elbow. As noted by Morrey, the elbow is a highly sensitive and “unforgiving joint.” All patients with traumatic elbow injuries should be counseled about some loss of motion, especially terminal extension. Anatomic restoration of the articular surface will help minimize the risk of posttraumatic arthritis. However, the exposure needed for adequate visualization to achieve this anatomic reduction often results in damage to the surrounding muscle and capsule, leading to scar tissue formation, heterotopic ossification, and a stiff joint.
The cause of posttraumatic motion loss of the elbow is often multifactorial; with causes being classified as either intrinsic or extrinsic. Intrinsic (intra-articular) causes are the result of the articular injury, such as cartilage loss, joint incongruity, or impingement. Extrinsic (extra-articular) causes are related to the periarticular soft tissue and bone, excluding the articular surface. Prolonged pain can produce both voluntary and involuntary guarding of the elbow during motion, eventually leading to contracture of the periarticular soft tissues and resultant stiffness. This pain mechanism is postulated as a possible explanation for stiffness occurring after even relatively minor trauma to the elbow.
Normal range of motion of the elbow joint as defined by the American Academy of Orthopedic Surgeons ranges from 0° (full extension) to 146° of flexion with 71° of pronation and 84° of supination. The goal of treatment when managing stiffness of the elbow is to restore functional range of elbow motion necessary to lead a normal life. Morrey and colleagues defined the functional range of motion of the elbow joint, necessary to carry out most ADLs, to be a 100° arc of motion, from 30° short of full extension to 130° of flexion, along with 50° of pronation and 50° of supination. Deficits in forearm pronation and supination are typically caused by a lesion at the radiocapitellar or proximal radioulnar joints, whereas deficits in flexion and extension are most often caused by ulnohumeral joint lesions.
The goal in the surgical management of periarticular elbow fractures is to anatomically reconstruct the elbow joint with a rigid construct that allows for early mobilization. However, this goal is often difficult to achieve, especially in patients with articular or metaphyseal comminution and associated soft tissue injuries or in elderly patients with osteoporosis. Fracture nonunion frequently results when early motion is attempted in the face of inadequate fixation. Unfortunately, prolonged immobilization to prevent failure of fixation can often lead to loss of elbow motion.
Distal Humerus Fracture
ORIF of distal humerus fractures is typically performed using a posterior approach, which may include an olecranon osteotomy. Loss of elbow motion is the most common sequela after ORIF of these fractures and is often observed even after stable fixation and appropriate rehabilitation. Dual plating, with either biplanar or parallel plating, is the standard fixation construct of intercondylar fractures of the distal humerus. The use of 2 plates on the distal humerus for complete articular fractures greatly assists the reconstruction of the triangle of stability of the distal humerus. Sanchez-Sotelo and colleagues treated 34 complex distal humeral fractures with their parallel plate technique and reported only 41% of elbows obtained at least 30° of extension and 130° of flexion. Other investigators have reported that about one-third of patients failed to regain functional arc of motion after ORIF of intercondylar fractures.
Terrible Triad Injury
Posttraumatic stiffness is a common complication after treatment of terrible triad injuries of the elbow. Three studies reporting outcomes after the surgical treatment of terrible triad injuries have documented a mean flexion-extension arc between 112° to 117° and mean rotation of 135° to 137°. A delay in treatment and a prolonged period of immobilization has been shown to lead to loss of elbow motion and poor outcomes. Broberg and Morrey similarly noted that immobilization for more than 4 weeks led to consistently poor results in their series of 24 patients. In the setting of a combined bony and ligamentous injury, a surgeon often has to decide between stability and mobilization. A stiff congruent elbow may be more amenable to interventions that result in clinical success than a mobile elbow with residual instability and incongruency.
Olecranon Fractures
Loss of motion is a common problem after fractures about the elbow but is rarely significant in patients with isolated olecranon fractures. A prospective randomized study comparing tension wiring and plate fixation of displaced olecranon fractures reported no difference in elbow range of motion between the 2 groups at final follow-up. The average loss of elbow extension was 10° in the tension band group versus 7° in the plate fixation group, whereas loss of flexion, pronation, and supination each averaged less than 5° in both groups. Patients with isolated injuries typically lose 10° to 15° of extension. However, in patients with associated fractures of the radial head, capitellum, or coronoid, or with a Monteggia fracture dislocation, the range of motion may be more severely compromised. Similarly, comminuted fractures and open injuries are also more likely to result in stiffness.
Management
The management of postoperative elbow stiffness begins with performing internal fixation that results in the elbow being rendered sufficiently stable to allow early rehabilitation and initiation of motion soon after surgery. Frequent follow-up and early identification of patients with elbow stiffness allows the surgeon to intervene before irreversible problems occur. The initial treatment of most patients with elbow stiffness is gentle passive and progressive active assisted stretching exercises under the guidance of a physical therapist. For patients whose motion has plateaued or not improved after a course of therapy, splinting is the next step. Dynamic hinged elbow splints with spring or rubber band tension may be useful to assist with deficits of elbow flexion. However, these devices often lead to spasm and co-contracture of antagonistic muscles, resulting in poor patient compliance. Turnbuckle braces (static progressive splints) are better tolerated and target either flexion or extension deficits. If contracture is present in both directions, the splint can be used in alternating positions.
In a prospective, randomized, controlled trial comparing the use of dynamic versus static progressive splints in the treatment of posttraumatic elbow contractures, Lindenhovius and colleagues showed no difference between the groups in the improvement of the arc of motion. The mean improvement of the flexion-extension arc was 40° versus 39° at 6 months and 47° versus 49° at 12 months, respectively, for the dynamic and static cohorts. Ten percent of the patients in the dynamic splinting group did not tolerate treatment and requested a change to the static splinting group before 3 months of use. A more recent systematic review of 232 patients with nonoperative management of elbow stiffness also concluded that both dynamic splinting and static progressive splinting were effective as a treatment of elbow stiffness, and that treatment should continue until patients stop making improvement in the elbow range of motion.
Continuous passive motion (CPM) machines may provide some benefit in the immediate postoperative period; however, these devices have a limited role in treating established contractures as they do not possess the mechanical advantage of static progressive splints to affect the end range of motion. If notable elbow stiffness remains despite splinting, further nonsurgical treatment is unlikely to be of benefit. The result of closed manipulation under anesthesia is unpredictable and may even produce loss of motion by producing inflammation and swelling with tearing of soft tissues, causing hemarthrosis and additional fibrosis.
If nonsurgical treatment fails, patients with diminished motion who can cooperate with the required rehabilitation program are candidates for surgical treatment. Assessment of the cause of the stiffness and the degree of functional impairment dictates the surgical approach to be used. For patients with little or no arthritis, soft tissue releases combined with the removal of impinging bone may be helpful. Resection of heterotopic ossification has been recommended after maturation of the bone, theoretically allowing the ossification process to complete and to avoid recurrence. A recent study looked at 2 groups of patients who underwent resection of heterotopic ossification at different time points. The early group had their resection at an average of 6.1 months, and the late group at 23 months. This study showed no difference in the recurrence rate (26% vs 28%) or range of motion or Mayo Elbow Performance score.
For patients without significant bony formation that limits motion, the direction of the greatest limitation of motion dictates the surgical approach and the capsular and tendinous structures to be released. Patients lacking flexion may benefit from ulnar nerve release and transposition, with release of the posterior band of the medial collateral ligament. Using this technique, Park and colleagues were able to improve elbow flexion in patients with posttraumatic stiffness from 89° to 124° on average. These investigators also noted that heterotopic ossification was present in 95% of patients who underwent surgery for elbow stiffness but was only identifiable on preoperative imaging in 54% of patients. The surgeon should have a high index of suspicion for the presence of heterotopic ossification in the patient with posttraumatic elbow stiffness, even if it is not apparent on radiographs. If this technique fails to achieve the desired amount of motion, a posterior capsulotomy can be subsequently performed through the same incision.
In patients lacking elbow extension, release of the anterior capsule is indicated in addition to resection of any bony blocks. Arthroscopic release of the anterior capsule has been shown to improve flexion contractures, with improvements in extension ranging between 17° and 21°.
Patients with moderate degenerative changes may be considered for limited bony arthroplasties, which include debridement arthroplasty or the Outerbridge-Kashiwagi ulnohumeral arthroplasty. Younger patients with severe degenerative changes can be treated with fascial interposition arthroplasty, with or without application of a joint distractor during the early healing period. For older or low-demand patients with advanced elbow arthritis, total elbow arthroplasty with a semiconstrained design can restore comfort and mobility.
Elbow arthroscopy has become an increasingly useful tool in the management of elbow stiffness following fracture fixation. Arthroscopic management of elbow stiffness is a viable alternative in certain patients, allowing smaller incisions, decreased soft tissue disruption, and, theoretically, easier rehabilitation. Soft tissue release techniques are being developed and refined. Mild impingement can improve with arthroscopic resection of osteophytes. In addition, elbow arthroscopy may also be beneficial when the status of the articular cartilage is uncertain by providing the surgeon with information needed to make decisions concerning the joint surface. Nguyen and colleagues reported their results of arthroscopic capsular release in 22 patients for the treatment of elbow stiffness. At a mean follow-up of 25 months, average elbow range of motion improved from 38°–122° to 19°–141°, with a 38° improvement in mean arc of motion. The arthroscopic treatment of elbow stiffness is technically demanding and the procedure does carry a significant risk of nerve injury. In a large series of 473 consecutive elbow arthroscopies, a transient nerve palsy occurred at a rate of 2.5%, with most patients regaining complete nerve function within 6 weeks. There were no cases of permanent neurovascular injury.
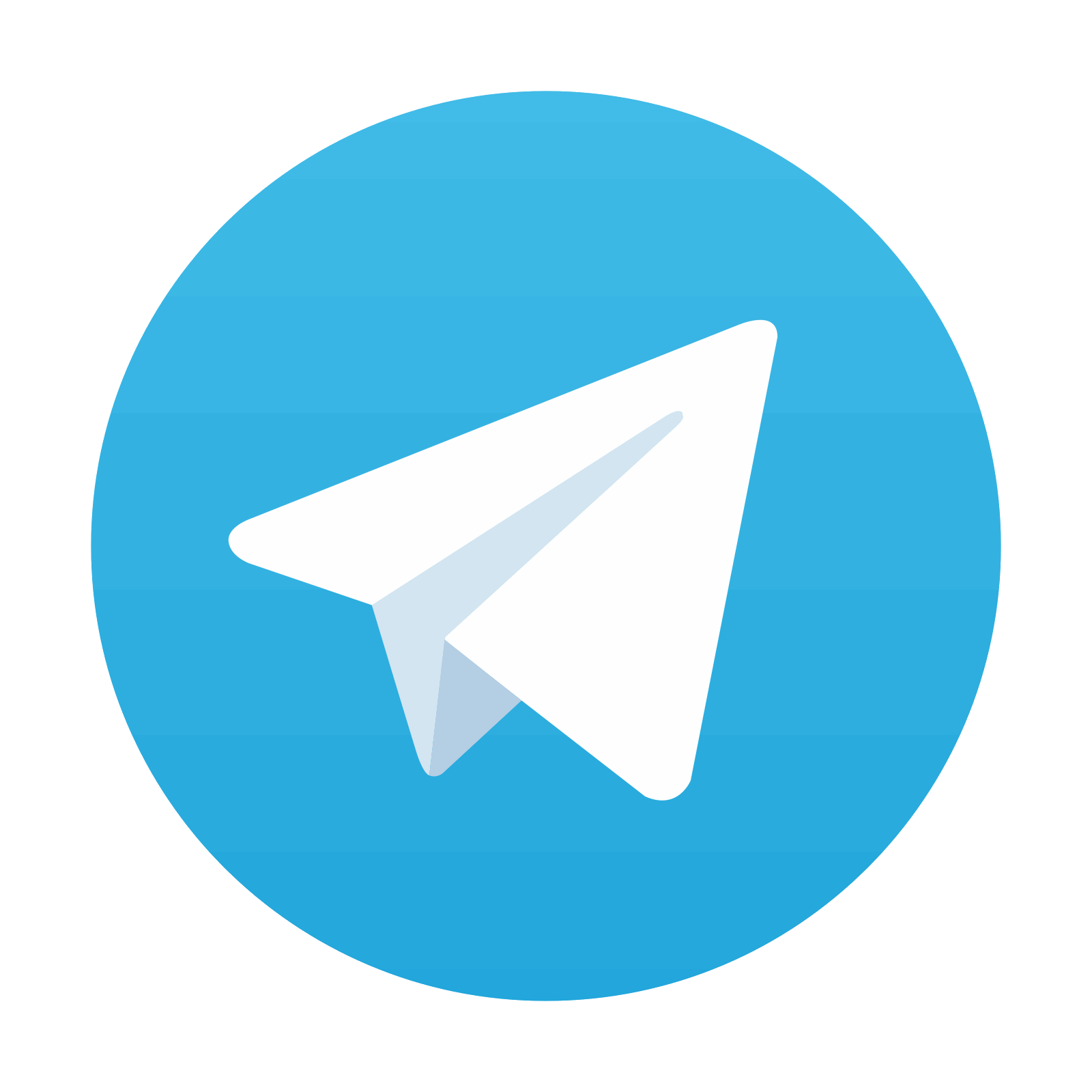
Stay updated, free articles. Join our Telegram channel

Full access? Get Clinical Tree
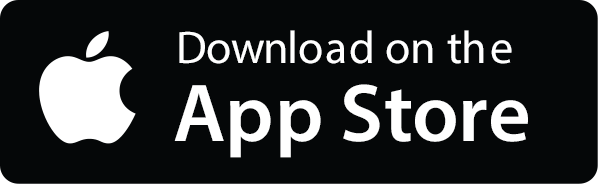
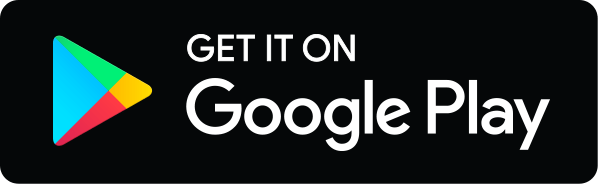