Anatomy and Biomechanics of the Elbow
James A. Johnson
Graham J.W. King
INTRODUCTION
The elbow is a highly complex structure consisting of the ulnohumeral, radiocapitellar, and proximal radioulnar joints. Together with a network of capsuloligamentous structures, elbow flexion and forearm rotation are permitted. Current implant replacement of the ulnohumeral articulation, the radial head, or both results in the restoration of joint motion pathways and function that can rival the native state for low demand activities. Despite numerous advances related to implant design, complications including joint stiffness, implant instability, and wear and loosening are not uncommon. This chapter begins with an overview of the anatomic features including bony and ligamentous structures that are relevant to joint function and motion. The general characteristics of load transfer and articular mechanics then are reviewed. The effect of the different implant designs on the kinematics and stability of the reconstructed ulnohumeral and proximal radius are subsequently described. The final sections discuss implant loading, fixation, and loosening.
ANATOMY
Normal Anatomy
Bone Anatomy (Osteology)
The distal humerus has two articulating portions: a spoolshaped trochlea, which articulates with the greater sigmoid notch of the proximal ulna; and a hemispherical capitellum that articulates with the concave surface of the radial head (Fig. 19-1).
The unique osseous shape and articular surface of the proximal ulna have important clinical implications (Fig. 19-1). The guiding ridge of the greater sigmoid notch runs longitudinally and articulates with the apex of the trochlear groove, whereas the medial and lateral portions articulate with the sloping surfaces of the trochlea. In flexion the coronoid process of the ulna locks into the coronoid fossa of the distal humerus, whereas the margin of the radial head is restrained by the radial fossa, further enhancing osseous stability. In extension, the tip of the
olecranon is contained by the olecranon fossa, increasing the osseous stability of the joint in this position. The complementary shape of the articular surfaces provides osseous joint stability throughout the range of motion.
olecranon is contained by the olecranon fossa, increasing the osseous stability of the joint in this position. The complementary shape of the articular surfaces provides osseous joint stability throughout the range of motion.
The radial head consists of a concave dish, which articulates with the capitellum, and an articular margin, which articulates with the lesser sigmoid (radial) notch of the ulna. Approximately 240 degrees of the radial margin articulates with the ulna, whereas the remainder is nonarticular and may be devoid of cartilage. Recent data have demonstrated that the transverse cross section of the radial head is not circular but somewhat elliptical in shape. Furthermore, the radiocapitellar dish is circular and offset from the neck of the radius (1). These nonsymmetric features have important implications in the reconstruction of the radial head with implants.
Soft-Tissue Anatomy (Capsuloligamentous Tissues)
The ligaments of the elbow are thickenings of the joint capsule. Medial and lateral thickenings form the medial and lateral collateral ligament complexes, respectively (2). The capsule attaches to the articular margins of the joint and blends with the fibers of the annular and collateral ligaments (Fig. 19-2). Anteriorly it includes both the coronoid and radial fossae, whereas posteriorly it includes the olecranon fossa. The anterior capsule has transverse and obliquely directed bands, which provide significant stability when taut in extension. The anterior capsule attaches to the distal tip of the coronoid process. The posterior capsule similarly becomes taut in flexion and may also have an important role as a static stabilizer in this position. The capsule allows maximal distension with the elbow in 70 to 80 degrees of flexion (3).
The medial collateral ligament (MCL) has three components: an anterior bundle, a posterior bundle, and a transverse portion (Fig. 19-2A). The humeral attachment of the MCL arises from the anteroinferior surface of the medial epicondyle. The anterior bundle is more prominent than the posterior bundle, and its insertion into the anteromedial portion of the coronoid and greater sigmoid notch of the ulna provides a significant mechanical advantage in controlling valgus forces. The anterior bundle has been subdivided by some authors into anterior, central, and
posterior bands (4, 5, 6). The posterior bundle is thin and fan shaped, inserting into the posteromedial margin of the greater sigmoid notch. The transverse ligament is variable in its definition and appears to have an insignificant role in stabilizing the elbow.
posterior bands (4, 5, 6). The posterior bundle is thin and fan shaped, inserting into the posteromedial margin of the greater sigmoid notch. The transverse ligament is variable in its definition and appears to have an insignificant role in stabilizing the elbow.
The lateral collateral ligament (LCL) complex consists of three parts: the annular ligament, radial collateral ligament, and lateral ulnar collateral ligament (7) (Fig. 19-2B). The origin of the LCL complex is on the lateral epicondyle of the humerus and is an important stabilizer throughout flexion. The annular ligament attaches to the anterior and the posterior margins of the lesser sigmoid notch. The funnel shape of the ligament stabilizes the proximal radius throughout the range of prosupination. The radial part of the collateral ligament blends with the annular ligament and is an important stabilizer of the radial head. Indirectly, through the annular ligament, this structure also stabilizes the humeroulnar joint (8). The ulnar part (also known as the lateral ulnar collateral ligament) extends from the lateral epicondyle to the crista supinatoris of the ulna and has been shown to be an important varus and rotational stabilizer of the elbow (7,9). Disruption of the lateral ulnar collateral ligament has been demonstrated to be an important cause of both acute and recurrent dislocation of the elbow (9,10).
Muscle Groups
The majority of the muscles crossing the elbow serve to rotate the forearm or flex and extend the wrist or fingers. Only a few muscles act primarily to move the elbow joint. By virtue of their joint reaction forces, all muscles that cross the elbow have the potential to provide increased joint stability by compressing the articular surfaces together. This stabilizing influence, however, is dependent on the position of the joint and the relative activity and line of action of the muscles acting across the articulation.
The primary elbow flexors are the biceps, brachialis, and brachioradialis muscles (11, 12, 13). The bicep has a smaller cross-sectional area than the brachialis; however, its mechanical advantage is greater because of its greater moment arm (13). The brachialis muscle has the largest cross-sectional area; however, it also has the poorest mechanical advantage of the elbow flexors. Its insertion into the anterior aspect of the proximal ulna suggests that it may have a role as an anterior buttress, stabilizing the elbow against posterior subluxation. The brachioradialis is an important elbow flexor despite its relatively small cross-sectional area. Arising along the lateral supracondylar ridge, it has the largest mechanical advantage of any of the muscles crossing the flexor surface of the elbow.
Elbow extension can be attributed almost exclusively to the triceps muscle. Consisting of three parts, the long, lateral, and medial heads, the triceps attaches onto and around the tip of the olecranon. By virtue of its close relationship to the axis of joint motion, the moment arm of the muscle is small, similar to that of the brachialis muscle anteriorly. The anconeus is a small muscle that is draped across the posterolateral aspect of the elbow covering the annular ligament and the radial head. Although its function remains unclear, its origin near the lateral epicondyle and insertion broadly on the ulna suggest it is an important dynamic constraint to varus and posterolateral rotatory instability of the elbow.
BIOMECHANICS
Natural Elbow
Kinematics and Stability
The elbow’s primary kinematic function is to position and stabilize the hand in space for bimanual activities. The elbow allows primary motions in flexion, extension, and pronation and supination. In view of the permitted motions in two degrees of freedom, the elbow has generally been described as a trochoginglymoid joint.
Flexion-Extension
The flexion-extension motion of the elbow has a range of approximately 0 to 140 degrees (2,35,36). Of this total arc only approximately 30 to 130 degrees are necessary to perform most activities of daily living (36). The motion pathway of elbow flexion-extension has been shown to approximate that of a loose hinge joint (37, 38, 39, 40, 41). The flexion-extension axis follows a line that can be drawn between the center of the capitellum and the center of curvature of the trochlear groove. However, the elbow joint is not a perfect hinge, and thus the flexion axis changes throughout the arc of motion (37,40). This is well illustrated by using screw-displacement axes, which show the instantaneous rotation and position of the axis throughout flexion (Fig. 19-3). (For a perfect hinge, all axes would coincide.) External landmarks that are useful in defining this axis are the anteroinferior aspect of the medial epicondyle and the center of the arc of curvature of the capitellum. On average, the axis of rotation is approximately 3 to 5 degrees internally rotated relative to the plane of the medial and lateral epicondyles and in 4 to 8 degrees of valgus with respect to the long axis of the humerus. It has been demonstrated that the locus of instant centers of rotation is small, moving less than 2 mm (37,38,42) throughout the arc of elbow flexion-extension. However, as shown from in vitro studies, the position of this axis will vary depending on forearm position (i.e., pronation versus supination) and whether the arm is moved passively (without muscle activity) or actively (37). An understanding of the patterns of elbow motion has fostered the development of articulated external fixators that are being increasingly used for reconstructive surgery and trauma. This has also allowed the development and clinical application of loose hinge total elbow arthroplasty design.
The carrying angle, which differs from the flexion axis, is another descriptor that is often used to describe the position of the elbow (43). For clinical purposes, the carrying angle is defined as the angle between the long axis of the humerus and the long axis of the ulna measured in the frontal plane with the elbow in the extended position. Considerable variation in the carrying angle exists between patients. Carrying angles are generally higher in women than in men. The average carrying angle for men has been reported to vary between 7 and 12 degrees; for women it is about 3 degrees greater (2).
Pronation/Supination
The range of forearm rotation as measured at the distal radioulnar joint is about 170 degrees, from approximately 90 degrees of supination to 80 degrees of pronation (35). To provide for this range, the radius rotates relative to the ulna at both the proximal and distal radioulnar joints. The axis of forearm rotation passes through the center of the radial head and near the fovea of the distal ulna (44,45) (Fig. 19-4). One study reported that this axis does not change with forearm rotation (46), although a study in 2002 suggested that it shifts slightly ulnar and volar in supination and radial and dorsal during pronation (47). The radius has been shown to move proximally with pronation and distally with supination (48), although another study has indicated that this may be subtle (47).
Forearm motion is clearly influenced by the morphology of the radial head. Direct measurements of the radial head have shown that the head is ovoid in shape; the maximal diameter exceeds the minimum diameter by an average of 2.5 mm (1,49). The radial head is variably offset from the axis of the neck on average by approximately 4 mm (1). In the case of an offset native head, a cam effect exists between the proximal radius and proximal ulna. During forearm rotation, as the radius rotates, and the edge of the radial head maintains contact with the lesser sigmoid notch, the radial shaft will move in the transverse plane, away from and toward the ulna (Fig. 19-5). As described later, reproducing natural forearm motion following implant reconstruction of the radial head is a challenge with currently available systems.
Stabilizers
Articular Surfaces
Proximal Ulna.
It has been shown that 80% of valgus loading is resisted by the proximal portion of the greater sigmoid notch, whereas 65% of varus loading is primarily resisted by the distal portion of the joint surface (50). The coronoid process of the ulna is a key stabilizer of the elbow. Failure to reconstitute this structure after fractures has been correlated with elbow instability and a poor functional outcome (51,52). Progressive removal of the proximal ulna produces a corresponding reduction in resistance to valgus loading (2). The coronoid process appears to be an essential osseous block to prevent posterior subluxation of the elbow joint, especially with the elbow in extension. Studies have shown that when greater than 50% of the coronoid is removed, axial loading results in an increased
posterior displacement, particularly at flexion angles greater than 60 degrees (53).
posterior displacement, particularly at flexion angles greater than 60 degrees (53).
Proximal Radius.
The articulation between the radial head and the capitellum is an important stabilizer to axial and valgus loading of the forearm. The radial head has been shown to be a secondary restraint to valgus elbow stability, with the MCL being the primary stabilizer (54). Biomechanical testing using a sequential sectioning protocol suggests that the radial head contributes about 30% of the valgus stability of the joint in both flexion and extension (55). Excision of the radial head with an intact MCL appears to have a small but significant effect on the valgus kinematics and stability of the elbow (55, 56, 57, 58). Complete disruption of the MCL was shown to produce an average 18-degree increase in varus-valgus laxity, and this increased to 36 degrees after radial head excision (58). In patients in whom the MCL or intraosseous membrane are injured, the radial head becomes the main stabilizer against valgus forces (59).
Distal Humerus.
Although the contribution of the articular surface of the distal humerus to the stability of the elbow has not been quantified, the circular shape of the trochlea with its deep groove is clearly important in stabilizing the ulnohumeral joint, just as the convex shape of
the capitellum is important in stabilizing the concave radial head.
the capitellum is important in stabilizing the concave radial head.
Capsuloligamentous Tissues
The Medial Collateral Ligament Complex.
The stabilizing influence of the MCL ligament has been widely studied. It has also been shown that distinct functions of the anterior and posterior bands of the anterior bundle exist, with the anterior band being more vulnerable to valgus loading in extension and the posterior band being more vulnerable in flexion (60). Numerous biomechanical investigations have demonstrated increased joint laxity after sectioning of the anterior bundle of the MCL (59, 60, 61, 62, 63), even with an intact radial head. Complete severance of the anterior bundle results in valgus and posteromedial rotatory laxity throughout the complete range of flexion (61). Clinically, the functional importance of the anterior bundle of the MCL has been confirmed and various ligament reconstructions have been developed and used to treat patients with a deficiency of this structure (4, 5, 6,55,59, 60, 61,63, 64, 65, 66, 67, 68, 69). The posterior bundle of the MCL has been shown to contribute little to the static stability of the elbow, with a moderate contribution to rotational stability (61). One study comparing the relative contributions of the three bands of the anterior bundle as well as the posterior bundle has shown that the central band of the anterior bundle provides the greatest resistance to varus-valgus loading (Fig. 19-6) (63).
![]() Figure 19-6 The maximum varus-valgus laxity (expressed as the mean ±1 standard deviation from eight specimens) is shown for the intact elbow and after sectioning of the structures of the medial collateral ligament. There was no difference between any of the sectioning sequences, with either the forearm pronated or supinated, until the central band was cut. (From Armstrong AA, Dunning CE, Faber KJ, et al. Single-strand ligament reconstruction of the medial collateral ligament restores valgus elbow stability. J Shoulder Elbow Surg 2002;11(1):65-71, with permission.) |
The insertion site of the MCL, particularly with respect to the axis of rotation, will affect the ligament tension and joint kinematics. Biomechanical studies have demonstrated an average 18% increase in the length of the anterior bundle from full extension to 120 degrees of flexion (64). It has been suggested that a guiding or isometric band exists within the central region of this bundle (4, 5, 6). Although perfect isometry may not occur throughout flexion, distinct bands of “near” isometry at the axis of rotation have been found (70).
Lateral Collateral Ligament Complex.
The LCL is the major stabilizing structure for both posterolateral rotational and varus stability (8,71, 72, 73, 74, 75). In the presence of an intact annular ligament, transection of both the radial and ulnar collateral ligaments at their humeral attachment appears necessary to induce posterolateral rotatory instability (8). Sectioning has little effect on joint stability in extension, although a fivefold increase in varus and rotational laxity of the elbow occurs at higher angles of flexion, even in the presence of an intact articulation (71). Other studies have indicated that sectioning of the entire ligament is necessary to produce varus-valgus laxity or a positive pivot shift test because sectioning of the radial collateral or lateral ulnar collateral in isolation is inconsequential (8,76) (Fig. 19-7). Reconstruction of the lateral ulnar collateral ligament restores varus and posterolateral
rotatory stability to the elbow and eliminates the lateral pivot shift both clinically and experimentally (77). Clearly preservation or repair of this ligament should be emphasized in patients undergoing surgical approaches to the lateral aspect of the elbow. The annular ligament appears to have an important contribution with respect to resisting varus and external rotation by virtue of its attachment to the radial collateral ligament (8,76).
rotatory stability to the elbow and eliminates the lateral pivot shift both clinically and experimentally (77). Clearly preservation or repair of this ligament should be emphasized in patients undergoing surgical approaches to the lateral aspect of the elbow. The annular ligament appears to have an important contribution with respect to resisting varus and external rotation by virtue of its attachment to the radial collateral ligament (8,76).
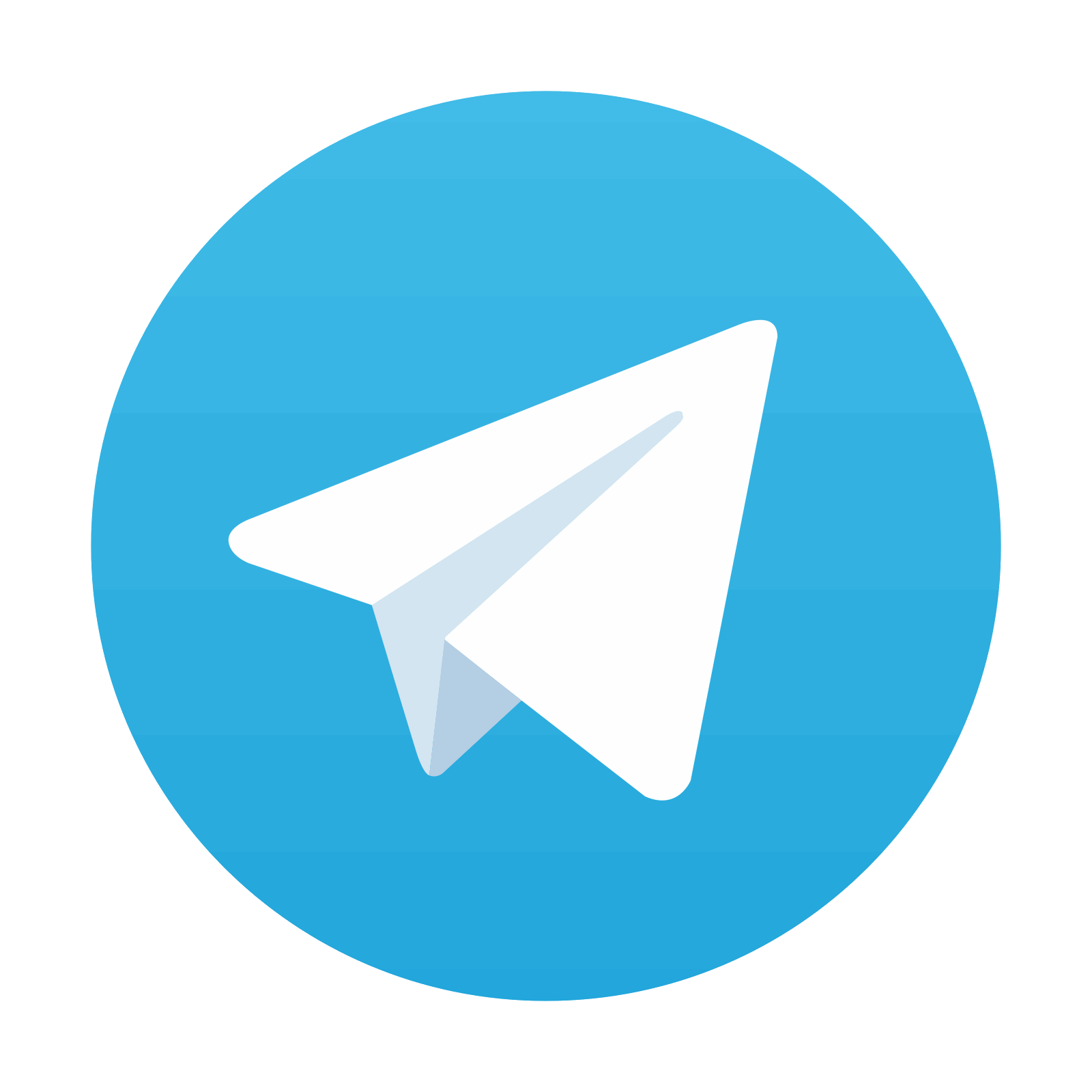
Stay updated, free articles. Join our Telegram channel

Full access? Get Clinical Tree
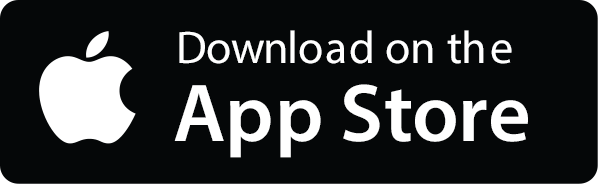
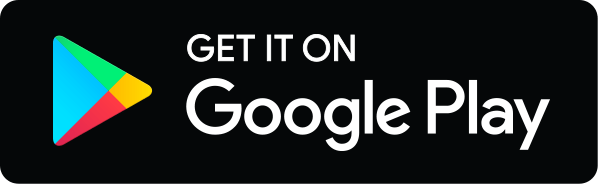
