Acknowledgment
We acknowledge James S. Starman, Mario Ferretti, Timo Järvelä, and Anthony Buoncristian, the authors of the previous edition, for their great contribution to this chapter.
Introduction
Anterior cruciate ligament (ACL) reconstruction is among the most common procedures performed in orthopaedics, with over 130,000 reconstructions performed annually in the United States alone. The ACL has therefore been extensively studied, and outcomes of ACL surgery have received considerable attention. Research on the ligament began with a search to determine its proper structure and function so as to best determine tunnel positions, graft choices, and fixation methods. In recent years, the focus has expanded to include postoperative rehabilitation protocols, changes in knee kinematics, and neuromuscular changes observed after surgery.
The ACL is composed of two bundles, termed the anteromedial (AM) and posterolateral (PL) bundles based off their relative insertion sites on the tibia. Each bundle possesses unique functional qualities that contribute to knee stability. When the ACL is injured, most often both bundles are involved. The primary goals after ACL reconstruction surgery are to restore knee stability and function, and prevent long-term joint degeneration.
Traditional ACL reconstruction focused on single-bundle reconstruction of the AM bundle. Using this technique, outcomes were satisfactory, with quoted success rates ranging from 69% to 95%. However, defining “success” after ACL reconstruction is difficult, as continued instability, inability to return to play, and early onset of posttraumatic osteoarthritis are frequent complications reported throughout the literature. A prospective study on ACL reconstructed patients 7 years after surgery revealed degenerative radiographic changes in 95% of patients, and only 47% were able to return to their previous activity level following ACL reconstruction. Several other studies have noted degenerative changes after ACL reconstruction, regardless of the chosen graft type. While trauma at the time of injury plays a role in inciting the degenerative cascade, surgical factors inevitably play a role as well. New studies are emerging in attempts to answer these questions.
In order to understand the injured ACL, one must first understand the anatomy and biomechanics of the normal ACL and its complex role in stabilization of the knee. Improved awareness of the anatomy and biomechanical properties of the normal ACL will lead to improvements in reconstruction techniques and outcomes. This chapter describes the normal anatomy and biomechanical contributions of the two bundles of the ACL.
Anterior Cruciate Ligament Anatomy
Historical Descriptions
The earliest known descriptions of the human ACL were made around 3000 bc and written on an Egyptian papyrus scroll. During the Roman era, Claudius Galen of Pergamon (199–129 bc ) described the ligaments of the knee and termed the ACL as “ligamenta genu cruciate.” In 1543 Andreas Vesalius completed the first known formal anatomic study of the human ACL in his book De Humani Corporis Fabrica Libris Septum .
For about 400 years, the ACL was considered to be a single homogeneous structure. Two bundles of the ACL were described for the first time in 1938 by Palmer. Despite other subsequent descriptions of the two-bundle anatomy by Girgis et al. (1975), the discovery did not become well known for many decades. Each author described an AM bundle and a PL bundle based on their relative tibial insertion sites; the same nomenclature is used today. Since then, it has become widely accepted that the ACL is composed of two bundles. The AM and PL bundles can be appreciated arthroscopically, particularly with the knee held in 90–120 degrees of flexion ( Fig. 1.1 ). Cadaveric dissection will also reveal the two bundles of the ACL ( Fig. 1.2 ). Although there is a considerable amount of individual variability with respect to the relative sizes of the AM and PL bundles depending on the type of study (i.e., fetal, arthroscopic, or cadaveric), all individuals with an intact ACL have both bundles.


More recently, Norwood and Cross (1979) and Amis and Dawkins (1991) have described a third ACL bundle termed the intermediate bundle. Although the two-bundle description may simplify the true anatomy and histology of the ACL, many studies have been based on this functional division, and this has been accepted as a reasonable way to understand the ligament. The intermediate bundle is most similar to the AM bundle in its anatomic and biomechanical properties. For the purposes of this chapter, it will be considered as part of the AM bundle.
Introduction
Anterior cruciate ligament (ACL) reconstruction is among the most common procedures performed in orthopaedics, with over 130,000 reconstructions performed annually in the United States alone. The ACL has therefore been extensively studied, and outcomes of ACL surgery have received considerable attention. Research on the ligament began with a search to determine its proper structure and function so as to best determine tunnel positions, graft choices, and fixation methods. In recent years, the focus has expanded to include postoperative rehabilitation protocols, changes in knee kinematics, and neuromuscular changes observed after surgery.
The ACL is composed of two bundles, termed the anteromedial (AM) and posterolateral (PL) bundles based off their relative insertion sites on the tibia. Each bundle possesses unique functional qualities that contribute to knee stability. When the ACL is injured, most often both bundles are involved. The primary goals after ACL reconstruction surgery are to restore knee stability and function, and prevent long-term joint degeneration.
Traditional ACL reconstruction focused on single-bundle reconstruction of the AM bundle. Using this technique, outcomes were satisfactory, with quoted success rates ranging from 69% to 95%. However, defining “success” after ACL reconstruction is difficult, as continued instability, inability to return to play, and early onset of posttraumatic osteoarthritis are frequent complications reported throughout the literature. A prospective study on ACL reconstructed patients 7 years after surgery revealed degenerative radiographic changes in 95% of patients, and only 47% were able to return to their previous activity level following ACL reconstruction. Several other studies have noted degenerative changes after ACL reconstruction, regardless of the chosen graft type. While trauma at the time of injury plays a role in inciting the degenerative cascade, surgical factors inevitably play a role as well. New studies are emerging in attempts to answer these questions.
In order to understand the injured ACL, one must first understand the anatomy and biomechanics of the normal ACL and its complex role in stabilization of the knee. Improved awareness of the anatomy and biomechanical properties of the normal ACL will lead to improvements in reconstruction techniques and outcomes. This chapter describes the normal anatomy and biomechanical contributions of the two bundles of the ACL.
Anterior Cruciate Ligament Anatomy
Historical Descriptions
The earliest known descriptions of the human ACL were made around 3000 bc and written on an Egyptian papyrus scroll. During the Roman era, Claudius Galen of Pergamon (199–129 bc ) described the ligaments of the knee and termed the ACL as “ligamenta genu cruciate.” In 1543 Andreas Vesalius completed the first known formal anatomic study of the human ACL in his book De Humani Corporis Fabrica Libris Septum .
For about 400 years, the ACL was considered to be a single homogeneous structure. Two bundles of the ACL were described for the first time in 1938 by Palmer. Despite other subsequent descriptions of the two-bundle anatomy by Girgis et al. (1975), the discovery did not become well known for many decades. Each author described an AM bundle and a PL bundle based on their relative tibial insertion sites; the same nomenclature is used today. Since then, it has become widely accepted that the ACL is composed of two bundles. The AM and PL bundles can be appreciated arthroscopically, particularly with the knee held in 90–120 degrees of flexion ( Fig. 1.1 ). Cadaveric dissection will also reveal the two bundles of the ACL ( Fig. 1.2 ). Although there is a considerable amount of individual variability with respect to the relative sizes of the AM and PL bundles depending on the type of study (i.e., fetal, arthroscopic, or cadaveric), all individuals with an intact ACL have both bundles.


More recently, Norwood and Cross (1979) and Amis and Dawkins (1991) have described a third ACL bundle termed the intermediate bundle. Although the two-bundle description may simplify the true anatomy and histology of the ACL, many studies have been based on this functional division, and this has been accepted as a reasonable way to understand the ligament. The intermediate bundle is most similar to the AM bundle in its anatomic and biomechanical properties. For the purposes of this chapter, it will be considered as part of the AM bundle.
Anterior Cruciate Ligament Development
ACL formation has been observed in fetal development as early as 8 weeks, corresponding to O’Rahilly stages 20 and 21. A leading hypothesis suggests that the ACL originates as a ventral condensation of the fetal blastoma and gradually migrates posteriorly with the formation of the intercondylar space. The menisci are derived from the same blastoma condensation as the ACL, a finding that supports the hypothesis that these structures function in concert. Another proposed mechanism of fetal ACL formation is that it forms from a confluence between ligamentous collagen fibers and fibers of the periosteum. Following the initial formation of the ligament, no major organizational or compositional changes are observed throughout the remainder of fetal development.
The two distinct bundles of the ACL begin to develop at 16 weeks of gestation ( Fig. 1.3 ). The fetal ACL has similar characteristics as the adult ligament, but differs in that the bundles are more parallel and the femoral origins are broader. Histologically, the fetal ACL is also more cellular and vascular. The two bundles are separated by a septum, similar to the adult ligament ( Fig. 1.4 ).


Histology of the Anterior Cruciate Ligament
The ACL is an intra-articular but extrasynovial structure, as two synovial layers envelop it. The ACL is a structure composed of numerous fascicles of dense connective tissue that connect the distal femur and the proximal tibia. Histological studies have demonstrated that a septum of vascularized connective tissue separates the AM and PL bundles ( Fig. 1.5 ). In addition, it has been shown that the histological properties of the ligament are variable at different stages in ACL development. At the time of fetal development, the ACL is hypercellular, with circular, oval, and fusiform-shaped cells. Later, in the adult, histology reveals the ACL to have a relatively hypocellular pattern with predominantly fibroblast cells with spindle-shaped nuclei. In addition to fibroblast cells, the ACL contains chondrocyte-like cells at higher concentrations around the tibial insertion, where “physiologic impingement” occurs.

Anatomy of the Anterior Cruciate Ligament
The ACL originates on the medial surface of the lateral femoral condyle (LFC), runs an oblique course within the knee joint from lateral and posterior to medial and anterior, and inserts into a broad area of the central tibial plateau. It is formed predominantly of Type I Collagen and secondarily of Type III Collagen. Proximally, the ACL receives its blood supply from the middle genicular artery, which feeds a synovial plexus around the ligament. Distally, the medial and lateral inferior genicular arteries supply the plexus but have a lesser contribution. Immunohistochemical analysis has demonstrated watershed areas exist around the insertion sites and along the anterior aspect of the distal third of the ligament.
About 1% of the ACL’s area consists of neural tissue supplied by branches of the tibial nerve. The neural tissue serves many functions within the ACL. The perivascular neural elements surround the vascular plexus and function in vasomotor control. Other nerve fibers function to transmit slow pain impulses. Surrounding the synovium are slowly adapting and rapidly adapting mechanoreceptors. The slowly adapting mechanoreceptors relay information about motion, position, and rotation of the joint, while the rapidly adapting mechanoreceptors detect changes in tension within the ligament. After ACL rupture, studies have demonstrated that the residual mechanoreceptors within the torn stumps may still function in proprioception. Further research is required to determine the extent of residual function.
The femoral and tibial insertion sites demonstrate a four-layered structure. The layers include ligamentous, fibrocartilaginous, mineralized cartilage, and a subchondral bone plate. This gradual transition prevents stress concentration at the insertion sites by dissipating the forces throughout the four layers.
The total intra-articular length of the ligament is approximately 22–41 mm (average: 32 mm) and varies by as much as 10% throughout a normal range of motion. The cross-sectional area of the ligament varies significantly throughout its course, from approximately 44 mm 2 at the midsubstance to more than 3 times as much at both its origin and insertion. While the overall cross-sectional area is constant during knee range of motion, the cross-sectional shape changes. At the tibial insertion, the ligament fans out into a “foot” region, allowing it to avoid impingement in the intercondylar notch during extension. This area demonstrates adaptive changes in which chondrocyte-like cells are mixed with the typical tenocytes in response to the “physiologic impingement.” After reconstruction, this “foot” region is unable to be restored, which can have implications for graft impingement and susceptibility for failure.
It is important to note that the bony anatomy is important as well. While the size of the ACL varies among people, the bony anatomy does as well, but not in a 1:1 proportion. Thus patients can have a relatively large ACL within a very small intercondylar space. As such, a small notch width index (ratio of the notch width to epicondylar width) has been linked to increased likelihood of ACL rupture.
Anatomy of Anteromedial and Posterolateral Bundles
Anatomic studies have characterized the individual contributions of both the AM and PL bundles to the overall ACL architecture. The AM bundle is approximately 38 mm in length. The PL bundle has been less well studied. Kummer determined an average PL length of 17.8 mm based on 50 cadaver dissections. Despite differences in length, the AM and PL bundles have a similar diameter. The narrowest aggregate cross-sectional area has been reported to be 36 mm 2 for females and 44 mm 2 for males. As the ligament approaches the insertion sites, it fans out. Harner et al. showed the footprints of the ACL are about 3.5 times larger than the midsubstance. This fact must be recognized during reconstruction.
Odensten and Gillquist described the femoral origin of the ACL as an ovoid area at an average of 18 mm in length and 11 mm in width. Within this area, the AM bundle occupies a position located on the proximal portion of the medial wall of the LFC, while the PL bundle occupies a more distal position near the anterior articular cartilage surface of the LFC ( Fig. 1.6A ). The lateral bifurcate ridge separates the origins of the two bundles. Harner et al. studied the origin and insertion of the AM and PL bundles using a laser micrometer system and concluded that each bundle occupies approximately 50% of the total femoral origin, with cross-sectional footprints of 47 ± 13 mm 2 and 49 ± 13 mm 2 for the AM and PL bundle, respectively. Mochizuki et al. contradicted these results and found the AM bundle origin was 1.5 times larger than the origin of the PL bundle, although a less sensitive methodology was used.

On the tibia, the insertions of the AM and PL bundles are located between the medial and lateral tibial spines over a broad area. The full ACL insertion has been described as an area measuring 9–13 mm (average 11 mm) in diameter medial-lateral and 14–20 mm (average 17 mm) in diameter anterior-posterior; not only does the size vary among individuals, but also the shape of the footprint differs as well. Within this area, the AM bundle insertion can be found in an anterior and medial position, whereas the PL bundle insertion is located more posteriorly and laterally (see Fig. 1.6B ). Posteriorly, fibers of the PL bundle are in close approximation to the posterior root of the lateral meniscus and, in some individuals, may attach to the meniscus itself ( Fig. 1.7 ). Similarly, the AM bundle may have attachments to the anterior horn of the lateral meniscus. The overall size of the tibial insertion is approximately 120% of the femoral origin. However, as is the case with the femoral origin, the two bundles share approximately equal tibial insertion site areas: the AM bundle occupies 56 ± 21 mm 2 , and the PL bundle occupies 53 ± 21 mm 2 .

Crossing Pattern
Based on their anatomical positions, the AM and PL bundles change alignment as the knee moves from extension to flexion. The femoral insertion sites are oriented vertically when the knee is in full extension, and the two bundles of the ACL are parallel in orientation ( Fig. 1.8 ). As the knee moves into 90 degrees of flexion, the AM bundle insertion site on the femur rotates posteriorly and inferiorly, in contrast to the femoral insertion of the PL bundle, which rotates anteriorly and superiorly; the femoral footprints become horizontally oriented ( Fig. 1.9 ). This change in the femoral footprint orientation causes the two bundles to twist around each other and become crossed. As the knee is flexed, the PL bundle can be seen anterior to the AM bundle at its femoral insertion ( Fig. 1.10 ).



Tensioning Pattern
The change in alignment of the AM and PL femoral insertion sites allows the ACL bundles to twist around themselves as the knee moves through a complete range of motion. This crossing pattern, along with the differences in the length of each bundle, has implications for the tensioning pattern of the overall ligament and each individual bundle. In a study by Gabriel et al., forces were measured in each bundle during an anterior tibial load of 134N over several flexion angles, as well as for a combined rotatory load of 10 Nm valgus and 5 Nm internal tibial torque. They showed that the PL bundle is tightest in extension (in situ force of 67 ± 30N) and becomes relaxed as the knee is flexed. When flexed and not on stretch, the PL bundle will shorten by 1.5–7.1 mm. Conversely, the AM bundle is more relaxed in extension, and reaches a maximum tension as the knee approaches 60 degrees of flexion (in situ forces of 90 ± 17N). Compared with extension, at 90 degrees of flexion, the AM bundle will stretch by about 3.3 mm. Several studies have corroborated these findings. This tensioning pattern can be observed grossly in cadaveric and arthroscopic views of the ACL ( Fig. 1.11 ). The PL bundle is also observed to tighten during internal and external rotation by about 2.7 mm. In summary, the ACL consists of two distinct bundles, the AM and PL bundles, and these bundles contribute synergistically to the dynamic stability of the knee.

Anatomic Considerations in Anterior Cruciate Ligament Surgery
The goal of ACL surgery is to reconstruct the ligament to have the same properties as prior to injury. While this may not be 100% feasible, certain principles must be employed to optimize outcomes. In order to quantify the femoral and tibial footprint locations, several methods have been developed using radiographic or anatomic landmarks. These include the quadrant method based off lateral x-rays, the clock description based off arthroscopic views, and the cylindrical coordinate system. Studies have tried to reference the ACL off other structures (i.e., the tibial insertion is 7–10.4 mm anterior to the anterior edge of the PCL in the flexed knee). Special guides have also been created to help create the femoral tunnel. Because all of these techniques attempt to generalize the footprints of the ACL, which vary person to person, they all have their limitations.
Anterior Cruciate Ligament Biomechanics
Historical Studies
The field of biomechanics has a long history, with the earliest known considerations dating back to Chinese and Greek literature around 400–500 bc . The first modern work in biomechanics was completed during the 1500s–1700s by well-known figures such as Galileo, DaVinci, Borelli, Hooke, and Newton. Orthopaedic biomechanics was initially advanced during the 1940s and 1950s by the work of Eadweard Muybridge, Arthur Steindler, Verne Inman, Henry Lissner, and A. H. Hirsch. Since the 1960s, the information learned from biomechanical studies in orthopaedics has been applied to refine clinical treatment approaches.
Anterior-Posterior Translation Control
The dynamic nature of the two bundles of the ACL during knee flexion demonstrates the complex role of the ACL in stabilization of the knee joint. However, initial biomechanical studies of the ACL focused mainly on its function as a restraint to anterior tibial translation. From this work, we know that the in situ forces of the ACL vary considerably during knee flexion. With a 110N anterior tibial load applied, the ACL demonstrates highest in situ forces between 0 and 30 degrees flexion, with a maximum at 15 degrees. In situ forces are at their lowest point between 60 and 90 degrees, with a minimum occurring at 90 degrees.
As mentioned earlier, recent studies have also evaluated the individual role of each bundle of the ACL in anterior-posterior translation. These studies have shown that the AM bundle has relatively constant levels of in situ force seen during knee flexion, whereas the PL bundle is more variable. The PL bundle sees higher in situ forces between 0 and 15 degrees of flexion but rapidly decreases with further flexion.
Rotational Stability
Recent studies have demonstrated that anterior tibial translation may not exclusively be responsible for improved patient outcomes after surgery, but rather rotational stability may play the larger role. While early studies were unable to indicate the exact rotational properties of the ACL, they did note the ACL fibers lengthened with internal rotation more so than external rotation. Furthermore, Andersen and Dyhre-Poulsen noted that in the absence of an ACL, the tibial plateau demonstrated increased internal rotation.
Within the last decade, a movement has started that has focused on studying the rotational-stabilizing function of the ACL. Using a cadaveric model, Gabriel et al. studied the in situ forces within the AM and PL bundles while a combined rotatory load of 10 Nm valgus and 5 Nm internal tibial torque was applied. The AM bundle demonstrated in situ forces of 30N at 15 degrees of flexion and 35N at 30 degrees of flexion. The PL bundle demonstrated greater changes with knee flexion. It saw forces of 21N at 15 degrees and 14N at 30 degrees. They concluded that both the AM and PL bundles contribute to rotational stability of the knee, each to varying degrees.
While these cadaveric studies paved the pathway for future research, they could not reproduce normal dynamics, including gravitational, inertial, or muscular forces. Within the last decade, a transition has taken place to study the ACL dynamically. Magnetic resonance imaging (MRI) studies using serial sequencing have studied in vivo kinematics of the normal ACL during weight bearing. Li et al. showed with increasing knee flexion during weight bearing, several changes occur. First, as the flexion angle increases, the axial rotation (“twisting”) of the ACL bundles increases as well. At full extension, the ACL is internally twisted by approximately 10 degrees. This internal twist increases to approximately 40 degrees with the knee at 90 degrees of flexion. Second, they noted the orientation of the ligament within the joint space changes with the flexion angle. As the knee flexion angle increased, so did the lateral angulation of the ligament. Therefore the ligament may possess a lateral force component, functioning to constrain internal tibial rotation.
In addition to biomechanical studies, recent studies using in vivo kinematics analysis have assessed the role of the ACL during various functional activities (i.e., walking, running, and cutting). Andriacchi and Dyrby studied the in vivo kinematics of normal and ACL-deficient subjects during four phases of walking and determined that an ACL-deficient knee is positioned differently than a normal knee. During the loading phase of gait, the ACL-deficient knee demonstrated increased internal rotation. A study of walking versus running and cutting in ACL-deficient patients demonstrated normal kinematics during walking. During running and cutting exercises, they noted abnormal anterior tibial translation and rotational movements compared with subjects who had an intact ACL.
In summary, the ACL is integral to rotational stability during both low- and high-demand activities. Both bundles of the ACL synergistically maintain normal tibiofemoral contact throughout the gait cycle by providing restraint to anterior tibial translation and conferring rotational stability.
Biomechanical Strength
Woo et al. demonstrated the ACL has an ultimate load to failure of 2160 ± 157N and stiffness of 242 ± 28 N/mm. This is relevant as it relates to various allograft and autograft options. According to one study, a 10-mm-wide patellar tendon graft has shown to have an ultimate load of failure of 1784 ± 580N and a mean stiffness of 210 N/mm, which is comparable to the natural ACL. A quadrupled hamstring autograft has a higher ultimate load to failure of 2422 ± 538N and a stiffness of 238 N/m, making it a reasonable graft choice as well. A caveat to the aforementioned is that studies have demonstrated that the graft may decrease in its tensile strength immediately after implantation; the tensile strength may take up to 12 weeks to reconstitute. This has implications on protection of weight bearing after surgery, and full incorporation may take up to 1 year or more after surgery.
However, like many tissues of the human body, the material properties of the ACL change with age. Biomechanical testing of cadaveric knees has shown that with age the ultimate load and stiffness decreases.
Biomechanics Considerations in Anterior Cruciate Ligament Surgery
The primary goals after ACL reconstruction surgery are to restore knee stability and function, and prevent long-term joint degeneration. Only a few decades ago, nonanatomic single-bundle ACL reconstruction was the most common surgical technique. In the last decade, there has been a movement toward anatomic ACL reconstruction, which restores the native footprints. Several studies have demonstrated the superiority of anatomic reconstruction compared with nonanatomic reconstruction. Perhaps the most important advantage after anatomic reconstruction is that the graft sees more of its native forces, which results in improved kinematics. Consequently, seeing more forces can lead to higher re-rupture rates.
Extending this concept that restoration of normal anatomy results in better restoration of knee kinematics led to the creation of double-bundle ACL reconstruction. Several studies have attempted to study anterior-posterior translation of the knee and rotational stability between single- and double-bundle reconstruction. Yagi et al. performed a cadaveric study comparing anatomic single-bundle versus anatomic double-bundle ACL reconstruction. They found that double-bundle ACL reconstruction demonstrated decreased anterior tibial translation at full extension and 30 degrees flexion, compared with the single-bundle technique. Additionally, when a combined internal tibial and valgus torque was applied at 15 and 30 degrees flexion, the double-bundle ACL reconstruction demonstrated increased rotatory stability. Yamamoto et al. performed a similar cadaveric study. They found double-bundle ACL reconstruction better restored the anterior tibial translation at 60 degrees and 90 degrees flexion when compared with the single-bundle technique.
Tashman et al. performed an in vivo kinematics analysis of native and single-bundle ACL-reconstructed knees, evaluating anterior-posterior tibial translation and rotation during downhill jogging. Single-bundle ACL reconstructed patients had fully restored anterior-posterior tibial translation, as compared with subjects with a native ACL, but were found to lack normal rotational kinematics. Because the single-bundle reconstruction is an approximation of the position of the AM bundle, it can be concluded that part of the rotational stability in the healthy knee is derived from the PL bundle.
While some studies have noted improvement in stability with double-bundle ACL reconstruction, due to differences in study design, surgical technique, graft types, and inclusion criteria, a final consensus has not been established. Clinically, at 2-years postoperatively, Ahldén et al. found no difference in anterior tibial translation via KT-1000 testing, pivot shift testing, range of motion, or subjective knee scores between anatomic single- or double-bundle reconstructions. Van Eck et al. performed a meta-analysis and found double-bundle reconstruction demonstrated superiority in anterior tibial translation and rotational stability based on clinical examination. Mascarenhas et al. noted the same conclusion in their meta-analysis, although noted clinical subjective outcomes were no different. With so many variables that influence outcome, additional high quality research is needed to delineate if a difference exists between the two techniques.
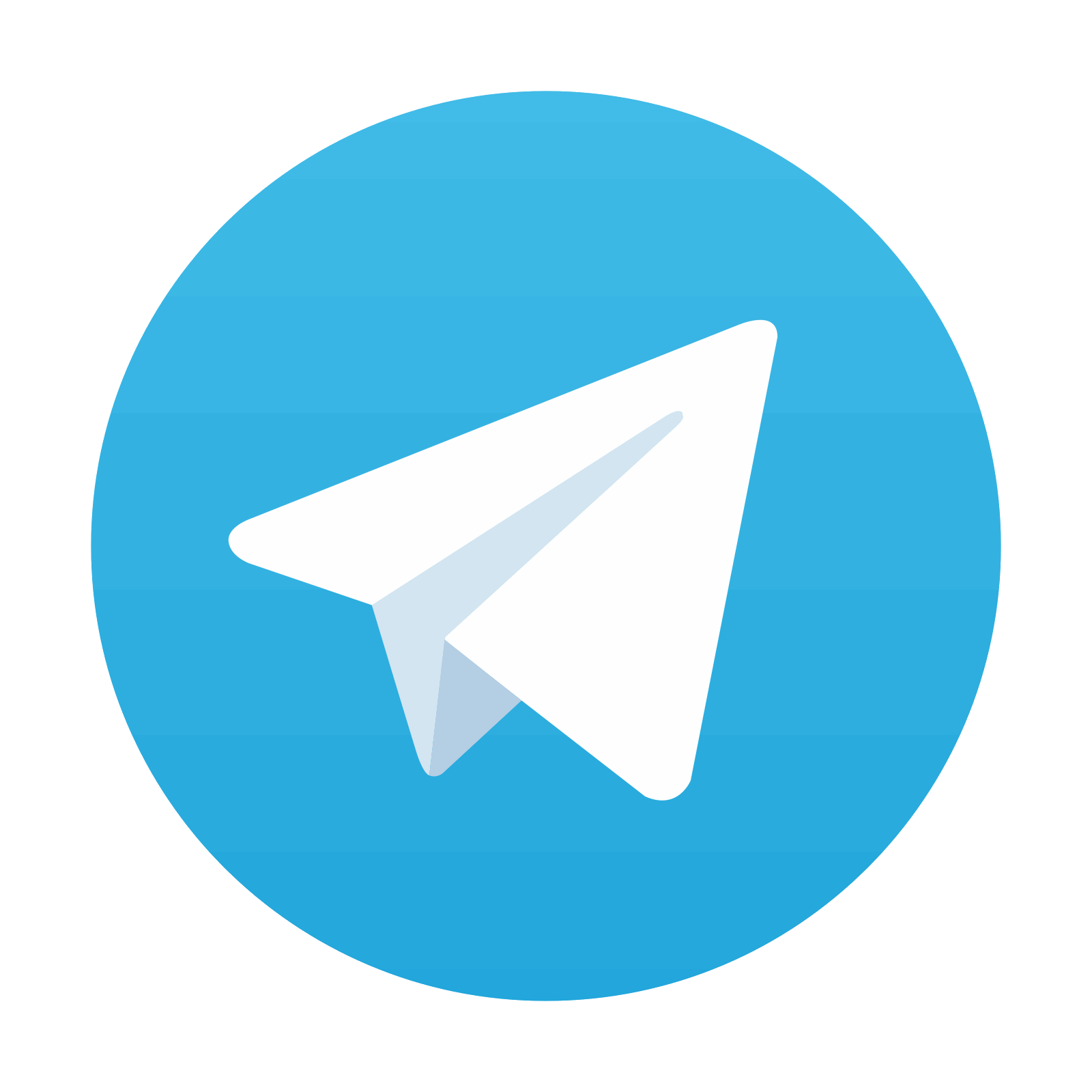
Stay updated, free articles. Join our Telegram channel

Full access? Get Clinical Tree
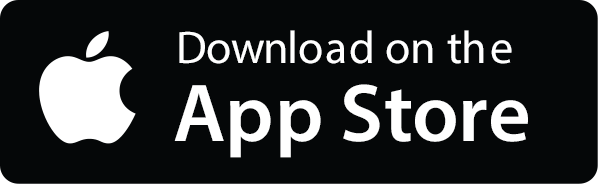
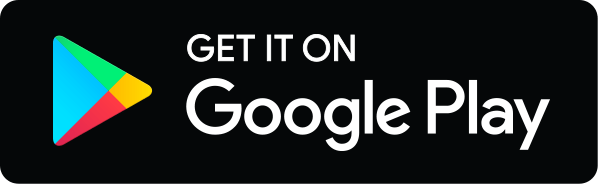