The Adaptive Immune Response
Most childhood autoimmune diseases are complex, multifactorial diseases. Through interactions with a defined genetic susceptibility, other, mostly unknown, environmental factors play a role in the pathogenesis. This interaction of genetic susceptibility and environment not only determines the onset of a disease but probably also its outcome. The adaptive immune system mediates key interactions at the interface between genetic background and the environment. Understanding adaptive immunity in autoimmune diseases is therefore crucial for our understanding of the immune pathogenesis of autoimmunity, and the generation of new therapeutic targets for drug development. In this chapter the key players in the adaptive immune system and their possible roles in pediatric autoimmune rheumatological diseases such as juvenile idiopathic arthritis (JIA), juvenile dermatomyositis (JDM), and juvenile systemic lupus erythematosus (JSLE) will be reviewed. Although much is now known about the interconnections between key players of the adaptive immune system, most data are from adult human subjects or mice; neither sets of data can be fully extrapolated to children or adolescents in whom the developing immune system and mechanisms are known to be distinct.
A group of closely interconnected immune cells including T cells, B cells, and antigen-presenting cells (APCs), together orchestrate the adaptive immune response. A primary function of the adaptive immune system is to offer protection against invading pathogens. The adaptive immune response is traditionally distinguished from the innate immune response by two distinctive properties: specificity and memory. After the initial innate immune response against an invading pathogen, T and B cells are generated that are specific for this antigen. Although the mechanisms of action differ between these different cell types, several fundamental features of adaptive immunity are shared by B and T cells. These include the ability to recognize pathogen components via clonal expression of unique cell surface antigen specific receptors; the ability to rapidly proliferate upon recognition of antigen, along with acquisition of cell lineage-specific immune functions; and the ability to persist after the infection is cleared, combined with the capacity to “remember” the pathogen or antigen, and respond more rapidly and vigorously upon reinfection (immunological memory). Thus, specificity is not lost after an immune response has waned, because so-called memory B cells and T cells are generated and maintained in the immune repertoire long term. This immunological memory ensures that a second encounter with the same antigen can be dealt with more rapidly and effectively. Immunological memory is the basis of one of the earliest and still one of the most effective immune-based interventions, namely vaccination.
The adaptive immune system is not an entity that stands or operates alone. Instead, it acts in a close association with the innate immune system (which is discussed in detail in Chapter 3 ). Innate immunity helps to initiate, drive, and steer the adaptive immune response. Several cell types including dendritic cells (DCs) and the recently recognized innate lymphoid cells (ILCs), exist as a bridge between innate and adaptive immunity. Thus the division between adaptive and innate immune cells is not black and white. Adaptive immune cells such as T cells may also carry out innate functions, whereas innate immune cells can act in a more specific way than previously was assumed. For example, while the triggering of a Toll-like receptor (TLR) most likely will lead to a proinflammatory response, it can also lead to temporary immune nonresponsiveness, depending on the timing, the location of the immune response, and local cofactors.
The close interaction between innate and adaptive immune cells is of special importance in chronic inflammatory diseases, such as JIA and other childhood autoimmune diseases. In these diseases it may be difficult, if not impossible, to separate entirely the effects of innate and adaptive immune activation. This is particularly the case when analyzing the immune response at the site of inflammation. Instead, in such “real-life” inflammatory environments, a continuous and dynamic interplay takes place, which can involve virtually all immune cells. Innate immune activation is extensively reviewed in Chapter 3 ; this chapter will focus on adaptive immunity, discuss the most important players of the adaptive immune response, and consider their importance and relevance for chronic inflammation as well as the cross-talk between adaptive and innate immunity.
Players in the Adaptive Immune Response and Their Roles in Autoimmunity
T Cells
T cells play a central part in the inflammatory response; both helper T cells and cytotoxic T cells are involved in most responses. T-cell immune responses typically involve an interplay of various effector mechanisms: the production of proinflammatory cytokines (including interferon [IFN]-γ tumor necrosis factor-alpha [TNF-α], and interleukin [IL]-17) and the expression of cytolytic effector molecules (including perforin and the granzymes A, B, and K), in addition to interactions with B cells, DCs, and other immune cells.
A significant feature of T-cell immunity is the ability of naïve T cells to undergo a program of proliferation and functional differentiation upon activation that results in a large pool of cells. All of these cells are capable of recognizing a particular antigen and have acquired the immune functions necessary to control and eventually clear infection. When infection is cleared, the majority of the expanded effector T-cell population dies; this leaves behind a small pool of long-lived memory T cells that can recognize the same antigen that triggered their initial activation. These memory T cells produce a broader array of immune molecules than naïve cells and in larger quantities, and are triggered at lower thresholds. Moreover, unlike naïve cells, they can respond to antigen without the need for further differentiation. These features, combined with persistence at a higher frequency, enable memory T cells to respond more rapidly when there is a secondary infection.
Evidence for the Role of T Cells in Autoimmunity
T cells are thought to play a central role in the immune pathogenesis of many human autoimmune diseases. The three key mechanistic areas that are thought to influence the T-cell arm of the adaptive immune system and its role in autoimmunity are as follows:
- 1.
Early development (commonly called central tolerance ). Antigens are presented to T-cell clones expressing specific T-cell receptors (TCRs) in the thymus. If there is a strong immune response, T-cell clones are autoreactive and undergo negative selection. Genetic predisposition to a high threshold of T-cell activation (i.e., less TCR signaling) may lead to a reduced selection against autoreactive clones. This, in turn, results in a predisposition to clinical autoimmunity.
- 2.
Activation/apoptosis ( peripheral tolerance ). Later in life, when naïve T cells are exposed to antigens, they respond by a similar mechanism of antigen–major histocompatibility complex–TCR signaling events. In this scenario, genetic predisposition to a low threshold of T-cell activation (i.e., stronger TCR signaling) may lead to an overexuberant immune response. Alternatively, high thresholds for T-cell activation might also lead to a predisposition of autoimmunity via a reduction of regulatory T-cell responses or a relative deficiency of apoptosis in responding effector cells.
- 3.
Polarization. After antigen exposure, naïve T cells differentiate into memory T cells of a specific subset (e.g., Th1, Th2, Th17), discussed in the following. Polarization into one or another subset is dependent on the balance of cytokines and transcription factors, as well as epigenetic modifications that regulate the distinct functional and phenotypic characteristics of T-cell subsets. Genetic factors also play a role in T-cell differentiation and may influence the threshold to develop into one subset or another. Consequently, there is an influence on the relative proportion of memory T-cell subsets and predisposition to clinical autoimmune disease.
The importance of T cells in autoimmune inflammation has been deduced from diverse sources, including experimental models, the study of human chronically inflamed tissues, and genetic studies. In JIA, various studies have underscored a potential role for T cells in the pathogenesis for most subtypes, with the exception perhaps of the systemic form of JIA, sJIA. The case for a role for T cells in JIA is based on multiple lines of evidence. At the onset of the disease, memory T cells with a proinflammatory phenotype are present at the site of inflammation. Among these T cells are cells with specificity for autoantigens, such as Hsp60 (heat shock protein 60 kD) and DnaJ. Secondly, the skewing of the TCR repertoire at the site of inflammation argues for a specific role of T cells instead of just being “spill over” from systemic inflammation. The fact that these skewed, highly oligoclonal T cells include cells specific for autoantigens has fueled the hypothesis that a pathogenic T-cell response against self-antigens lies at the heart of JIA. However, the picture is not entirely straightforward. For example, in many animal models of arthritis, T cells orchestrate the disease-related immune responses in such a way that they can either protect from arthritis or induce it. In other models, however, arthritis can be induced in the absence of T cells, underscoring that it is not T cells alone that determine arthritis. More “circumstantial” evidence on the importance of T cells in JIA comes from genetic studies.
Genetics That Implicate T-Cell Pathways
A prominent genetic region that is involved in determining risk for human autoimmune diseases is the human leukocyte antigen (HLA) region located on chromosome 6, also known as the human major histocompatibility complex (MHC). MHC molecules can be divided into MHC class I and MHC class II. HLAs A, B, and C code for MHC class I molecules. MHC class I molecules present peptides such as viral peptides from inside the cell to CD8 + T cells. On the other hand HLA DP, DM, DO, DO, DQ, and DR code for MHC class II molecules, which can present processed antigenic peptides to CD4 + T cells.
In most complex autoimmune diseases a strong genetic association exists with the HLA region. This link with HLA is further strong evidence for an essential role for T cells in the etiology of autoimmunity. It still remains mostly undefined how this relationship translates into a mechanism, with a few notable exceptions. One possibility is that negative selection in the thymus (see below) in the presence of certain MHC alleles is affected in such a way that it increases the chance of T cells specific for certain MHC–self-peptide combinations to escape negative selection in the thymus.
In both rheumatoid arthritis (RA) and JIA, the strongest genetic associations lie in HLA regions. The HLA associations in some subtypes of JIA (such as oligoarticular JIA), are with different alleles than those in RA ; in contrast, juvenile-onset polyarticular JIA shares MHC class II allele risk associations closely with RA. Although HLA is the most prominent susceptibility factor in JIA, it only explains around 10% of the total variation of JIA susceptibility. In addition, a large number of non-HLA candidate genes have been found to be associated with JIA, such as genes coding for PTPN22, PTPN2, IL-2RA, MIF, SLC11A6, WISP3, and TNF-α. A genetic study of almost 3000 cases of JIA using the Immunochip platform confirmed associations of a total of 16 non-HLA loci at genome-wide significance, including IL-2/21, STAT4, IL-2RA, IL-2RB, RUNX1, ERAP2, and others. These and other genetic associations point to a central role for the immune system, and more specifically the adaptive immune system in the pathogenesis of JIA. For example, multiple studies have revealed associations with genes related to the IL-2 and IL-2R pathways, which are a crucial for T-cell differentiation and function, again underscoring the importance of T cells to JIA immune pathogenesis.
T-Cell Subsets and Effector Mechanisms
T cells can be grouped into different subsets, based on their phenotype and effector functions. CD8 + T cells, also called cytotoxic or killer T cells, express the surface molecule CD8 and are classically important for defense against intracellular pathogens and viruses. They recognize proteins derived from foreign antigens or self-antigens that are expressed on the cell surface of APCs in the context of MHC class I molecules. A main effector mechanism is to kill target cells through the release of various cytotoxic proteins such as granzymes and perforins. CD4 + T cells, also called T-helper (Th) cells , are characterized by surface expression of CD4, and recognize exogenous and self-antigens presented in the context of MHC class II molecules on professional APCs.
A significant feature of T-cell biology is the enormous functional plasticity of naïve T cells. An example of T-cell functional plasticity is found when naïve helper T cells are activated and may differentiate into distinct functional types, mainly defined by the soluble effector molecules they secrete. Initially three Th cell subsets were recognized: Th0 cells, Th1 cells, and Th2 cells. Th0 cells are undifferentiated naïve cells, whereas Th1 cells are characterized by the production of IFN-γ and IL-12. Th1 cells are responsible for the delayed type hypersensitivity (DTH) reaction and play a role in the normal adaptive immune response against various (intracellular) microorganisms. Th2 cells provide crucial help for B cells and antibody production. They play a vital role in the defense against parasitic infections and are related to allergic disease. Whereas Th1 cells produce proinflammatory cytokines such as IFN-γ, Th2 cells may have a more antiinflammatory phenotype, and produce predominantly IL-4, IL-5, and IL-10.
Because of their apparently opposing cytokine profiles, Th1 and Th2 cells were long thought to be in dynamic balance. However, with increasing understanding of T-cell biology it is clear that this model was oversimplified. Many other T-cell subsets have now been defined, both in mice and in humans, which are unique and significant in their own way, including Th17, regulatory T (Treg) cells, TFh (follicular Th cells), Th22, and Th9 cells ( Table 4-1 ). To date, at least nine distinct Th subsets can be distinguished that are either mostly proinflammatory (such as Th1 and Th17 cells) or mostly antiinflammatory (such Treg and Treg type 1 [Tr1] cells). Many of these distinct subsets have been shown to be driven by specific transcription factors ( Table 4-1 ).
TH SUBSET | GATE KEEPER TRANSCRIPTION FACTOR | CYTOKINES INVOLVED | RELEVANCE IN HUMANS | PUTATIVE-RELATED DISEASES |
---|---|---|---|---|
Th1 | Tbet, STAT4 | IFN-γ, IL-12 | Protection against microorganism DTH | Autoimmune diseases |
Th2 | GATA-3 | IL-4, IL-5, IL-13 | Protection against parasites, allergy, B-cell help, class switching | Allergy |
Th3 | Unidentified | Transforming growth factor-β (TGF-β) | Mucosal tolerance | Unidentified |
Th17 | RORC | IL-17 (IL-6, TGF-β) | Autoimmune diseases | |
Treg | FOXP3 | (consumption of) IL-2 | Regulation of inflammation | Autoimmune diseases, JIA |
Tr1 | Unidentified | IL-10 | Regulation of inflammation | Diabetes |
Tfh | Bcl6 | IL-21 | Isotype switching and B-cell memory | Variety of inflammatory diseases |
Th9 | Unidentified | IL-9, TNF-α, and granzyme B | Protection extracellular pathogens | Psoriasis |
Th22 | Unidentified | IL-22 | Protection and damage control | Variety of inflammatory diseases |
The tailoring of helper T-cell responses into distinct functional lineages is a consequence of integration of multiple signals that are present during initial T-cell activation, including local cytokines, other ligand interactions, and metabolic signals. Indeed, there is heterogeneity of effector function within a responding T-cell population, and no one immune response is uniquely represented by a single Th subset. In fact, there is tailoring of the total T-cell population such that a particular subset may be overrepresented, but many immune responses will involve several types of effector T cell.
T-Cell Subsets in Autoimmunity
In the context of autoimmunity much recent attention has focused on Th1 and Th17 cells. Both are considered to be important mediators of a detrimental immune response in autoimmunity. A pathogenic role for Th17 cells has been implicated in JIA as well as several other autoimmune diseases, such as psoriasis and multiple sclerosis. Of special interest is the fact that there is a connection between these proinflammatory Th17 cells and regulatory FOXP3+ Treg cells. This interrelationship and its role in childhood autoimmunity is further discussed below. Deciphering this complex relationship is a prime objective of future research, as it will be indispensable for the development of cell-based therapy in autoimmune diseases.
Regulatory T Cells
On the other end of the spectrum from inflammatory Th cells, are the so-called regulatory T cells, which have gained significant interest over the past decade. Various types of Treg cells are now recognized, of which FOXP3 expressing Treg, and IL-10–producing Treg type 1 cells (Tr1) are among the most studied in humans. FOXP3+ Treg cells are characterized by the expression of the transcription factor FOXP3 and can either be directly derived from the thymus (natural Treg) or induced in the periphery (adaptive Treg). In experimental models, the presence or absence of FOXP3-expressing Treg cells can determine the presence or absence of disease. In humans, gene mutations in FOXP3 lead to immunodysregulation polyendocrinopathy enteropathy X-linked syndrome (IPEX), a fatal multisystem inflammatory disease with onset early in life, underscoring that in humans Treg cells are also crucial for maintaining immune tolerance. On the other hand, in most human autoimmune diseases there seems no quantitative deficiency of Treg cells. Instead, an increased number of Treg cells is often found at sites of inflammation, such as the synovial fluid in JIA. However, clearly these are insufficient or unable to suppress inflammation. Whether the Treg cells at the site of inflammation are deficient in function is still a matter of debate.
Also crucial for maintaining peripheral tolerance are Tr1 cells. They are characterized by the production of IL-10 next to other cytokines such as IL-4 and IL-7, and the expression of CD49b and LAG-3. They were first found in studies related to stem-cell transplantation and are now being developed for cell therapy in transplantation setting. However, little is known about their role in JIA. To further complicate our understanding of Treg cells, different functional states can exist within the various subtypes of regulatory cells. For example, FOXP3+ T cells can have a phenotype that resembles Th1, Th2, or Th17 cells and in some circumstances have been shown to produce inflammatory cytokines.
T-Cell Plasticity
It is now clear that there is considerable plasticity in the T-cell system such that both Th effector and regulatory cells of one type may change into another functional type. For example, evidence from both mouse and human autoimmunity has been demonstrated that Th17 cells, when exposed to an inflammatory environment containing IL-12, may convert to double-producing Th1/Th17 or even Th1 cells. These “ex-Th17” cells have been shown to produce high levels of the proinflammatory granulocyte-macrophage colony-stimulating factor (GM-CSF) in JIA at the inflamed site.
Similarly, considerable evidence suggests that Th17 and Treg cells may interconvert depending on local cytokines. Th17 cell and adaptive Treg cell differentiation are both dependent on transforming growth factor (TGF)-β. In mice the combination of TGF-β with proinflammatory cytokines IL-1β and IL-6 allows the differentiation toward a Th17 phenotype, whereas TGF-β alone induces adaptive Treg cells. Several other factors may influence the balance between Th17 and Treg cells, including IL-2 and retinoic acid (a derivative of vitamin A). In both human and murine studies, data suggest that Treg cells may convert to Th17 cells under inflammatory conditions.
Thus, keeping a healthy immune balance and how this balance is altered in inflammation involves a highly interactive system of communicating T cells that have the ability to adapt easily to a changing environment. This adaptability may be crucial in allowing normal immune responses, because a proinflammatory immune response mounted as a defense against an invading microorganism would lead to serious tissue damage if not controlled properly. However, it will be vital to fully understand what regulates such T-cell plasticity if T cells are to be used in novel cell therapy approaches (for example, infusion of Treg cells).
B Cells and Antibody Production
B cells are crucial players in the adaptive immune response. They are an important part of the defense against pathogens through their capacity to produce antigen-specific, protective antibodies. Moreover, in the constant interaction between cells of the adaptive immune system, B cells are also potent APCs and as such are pivotal for T-cell activation and creating the right immunological environment for the optimal specific immune response. There is much evidence for a role of B cells, and the autoantibody-producing plasma cells they mature into, in the pathogenesis of autoimmunity. In various human autoimmune diseases, hypergammaglobulinemia is a prominent laboratory feature of active disease. In diseases such as systemic lupus erythematosus (SLE) and JDM, the presence of specific autoantibodies is directly related to disease activity or disease subtype. These antibodies are increasingly used as biomarkers to drive clinical care decisions (see Chapters 23 and 26 ). In both adult and childhood inflammatory arthritis, the presence of autoantibodies, such as antinuclear antibodies (ANAs) in JIA, or rheumatoid factor and anti-cyclic citrullinated peptide (anti-CCP) in RA, are important biomarkers that help both to classify distinct groups of patients, and also serve as prognostic and diagnostic markers (see Chapter 17 ). Furthermore, these autoantibodies may be present before disease onset. Thus, the fact that anti-CCP antibodies increase the risk for subsequent development of RA, and anti-double stranded DNA (anti-dsDNA) antibodies increase the risk for SLE, indicates there is a direct association between B cells and plasma cells and the development of autoimmune diseases. However, the exact mechanisms through which most autoantibodies contribute to the pathological inflammatory responses or tissue damage in these diseases are still unclear.
In addition to being a source of autoantibodies, it is increasingly clear that B cells and plasma cells also have functional effector diversity and therefore may play important proinflammatory roles in disease through the production of cytokines. The robust clinical efficacy of B-cell depletion by anti-CD20 therapy in many systemic autoimmune diseases (RA, SLE, and myositis) underscores the important role of B cells in autoimmunity (see also Chapter 13 ).
Regulatory B Cells
Recently, the recognition of B cells with an antiinflammatory phenotype has gained attention. Studies in both mice and humans have demonstrated that B cells can have immunosuppressive functions. These so-called regulatory B (Breg) cells have the potential to actively regulate an ongoing immune response, for example, through the production of IL-10 and, more recently demonstrated, IL-35. IL-10–secreting B cells have been shown to promote disease remission in mouse models of autoimmune disorders. Human B cells may also produce IL-10, and there is increasing evidence to support the idea that human IL-1–producing B cells can also inhibit immunity. Human Breg cells have been shown to be within a population of transitional B cells, defined by the phenotype CD19 + CD24 hi CD38 hi and have been shown to be defective in human autoimmune diseases such as lupus.
Dendritic Cells
DCs are key regulators of adaptive immunity as part of the first-line defense against microorganisms. They are potent initiators of immune responses as well as mediating immune tolerance (see the next section). First recognized by Steinman (for which he received the Nobel Prize in 2011) and others more than 40 years ago, DCs form a bridge between innate and adaptive immunity. They are part of lineage of cells that include Langerhans cells, plasmacytoid dendritic cells (pDCs) and monocyte-derived dendritic cells (mDCs); the latter are also referred to as classical dendritic cells or cDCs. DCs can identify and kill potential harmful microorganisms while also processing self-antigens. In doing so they can attract and direct other immune cells and create the proper microenvironment for an effective immune response.
Dendritic Cells in Autoimmunity
DCs may drive both highly inflammatory as well as regulatory responses. The resulting effector response generated will depend in part in levels of costimulatory molecules, cytokines produced by DCs and the strength of APC/T-cell interaction. The mechanisms of action of tolerogenic DCs is still not known, but the production of cytokines (such as IL-10 and TGF-β) and the increased prevalence of tolerogenic versus stimulatory membrane-bound coreceptors appears to have a role. Simultaneously, Treg cells can downregulate the expression of costimulatory molecules (CD80/86) on DCs, through the action of CTLA4, which serves to inhibit antigen presentation to effector T cells.
Much evidence from experimental models indicates that DCs play a key role in autoimmunity. Thus the deficiency of TGF-β signaling specifically in DCs leads to experimental autoimmune colitis, whereas DC-specific knockout of genes associated with human autoimmunity on genome-wide association studies (GWAS), such as Blimp1 and TNFAIP3, leads to autoimmune phenotypes in experimental animals. TNFAIP3, also known as A20, is a ubiquitin-modifier that has a profound antiinflammatory effect, by restricting DC-mediated immune activation, in part through modulating nuclear factor κB (NF-κB) signaling. Interestingly, in the TNFAIP3-deficient mouse model, the disease mimics inflammatory bowel disease, enthesitis, and ankylosing spondylitis (AS), conditions that frequently also occur in humans. On the other hand, DC-l specific knockout of Blimp1 leads to the presence of humoral autoimmunity with a lupuslike serology.
In humans, pDCs can induce T cells to differentiate into suppressor or Treg cells, but they may also be the source of type 1 interferons thought to play a role in SLE and JDM. Perhaps their most important role in steering the adaptive immune response, whether toward effector or regulatory functions, is their capacity to process antigens and present them to T cells. Because of their central role in immune response initiation and regulation, DCs are important targets for innovative forms of immune therapy.
Maintaining Immune Tolerance and Balance in the Adaptive Immune System
Central Tolerance
Maintaining the balance of the adaptive immune system requires an extensive system to keep potential autoreactive T and B cells under control. Prevention of the emergence of autoreactive cells takes place both centrally (at the selection of the repertoire) and in the periphery. In the thymus, T cells are generated that need to have a diverse range of TCRs so that the final repertoire has the potential to cope with an enormous range of different foreign antigens. Similarly, it is crucial that T cells with a TCR with a high affinity for self-antigens are eliminated from the repertoire. This negative selection is dependent on the local expression of tissue-specific antigens in the thymus. This process is controlled by the autoimmune regulator protein (AIRE). Absence of AIRE leads to severe autoimmunity both in animal models and in humans (autoimmune polyendocrinopathy-candidiasis-ectodermal dystrophy syndrome [APECED]) (see Chapter 46 ).
Peripheral Tolerance
Central selection processes are not absolute and not sufficient to prevent harmful autoreactivity. Therefore the immune system harbors other control mechanisms in the periphery. These include the presence of numerous regulatory mechanisms that can be tissue specific and/or cell specific. Of the cellular mechanisms, Treg cells are the best studied, but an increasing body of evidence supports a role for Breg cells, as discussed previously. It is clear that DCs also play a key role in maintaining tolerance in part by the generation of Treg cells. In addition, immune control mechanisms are not mediated only by adaptive immunity. Innate immune cells, such as neutrophils, can also have a regulatory, suppressive effect on a potential damaging immune response.
The Inflammatory Response Out of Control in Autoimmunity
Identification of “Self” Versus “Nonself”
The conventional vision of the tolerance process is somewhat dichotomous. It is based on the concept that there is a clear partition between self and nonself, and that the immune system has evolved to ignore self and react against environmental challenges. However, in reality the process of the ontogeny of the most sophisticated part of the immune system, T-cell adaptive immunity, is entirely shaped on recognition of self-antigens, presented in the thymus in the context of self-MHC to immature T cells. T cells whose TCR binds self-antigens with high affinity are deleted, as they would be highly self-reactive, whereas T cells whose TCR binds self-antigens at medium affinity survive and will comprise the mature T-cell repertoire. In the periphery, self-peptides are constantly recognized by the same T cells, and such interactions contribute to maintenance of T-cell clones throughout life. Hence, recognition of self is a necessary and ever-occurring phenomenon for the immune system. Autoimmune disease occurs when the delicate balance between recognition and tolerance tilts toward reactivity. This is most likely to occur in the microenvironment where an autoimmune reaction originates, perhaps in combination with inflammatory triggers such as local infection or tissue damage. Loss of tolerance is the outcome of environmental, stochastic, and epigenetic elements shaping the cross talk between adaptive and innate immunity.
Cross-Talk Between Adaptive and Innate Immunity in Disease
A frequent misconception of the immune system is that it is compartmentalized, with its various components dissected and isolated in “waterproof” compartments. This approach may simplify the sharing of knowledge about individual components of the immune system, and the identifying of potential targets for therapeutic intervention. However, it is important to underscore that the immune system is dynamic and its individual elements profoundly intertwined. In particular, interference in any one individual pathway has effects far beyond the intended target, and eventually involves many components of the immune response. For example, it is evident that JIA, like other autoimmune arthritides, is multifactorial, and several immune pathways contribute etiologically and pathogenetically.
Th17 cells and their relationship to Treg cells in disease
Th17 cells express the transcription factor retinoic acid receptor-related orphan nuclear receptor C (RORC) and secrete the proinflammatory cytokine IL-17. Th17 cells from inflammatory sites such as the joints of children with JIA also frequently produce other cytokines including TNF, GM-CSF, and others. As Th17 cells and Treg cells share a close relationship, understanding how these cells interact and what consequence this may have on JIA will help to further understand this complex disease. Patients with active JIA have increased numbers of Th17 cells and reduced numbers of Treg cells compared with healthy controls in peripheral blood. However, at the inflamed site, both these subsets are highly enriched, and there is a reciprocal relationship between Th17 and Treg cells. Interestingly, the subset of Treg cells that can also produce IL-17 (identified by the surface marker CD161) is highly enriched in the synovial compartment in JIA and correlates with disease activity. In addition to a potential imbalance between Th17 cells and Treg cells, it appears that the effector T cells within the synovial compartment may be resistant to Treg-mediated suppression. Therefore, Th17/Treg imbalance, Treg cells that have switched to become proinflammatory, and the resistance of Th17 cells to Treg-mediated suppression may all contribute to the pathogenic process in JIA. Key drivers of these switches, and how they may be programmed in terms of epigenetic and transcriptional modulation, remain unknown. Data from mouse models and adult RA suggest that anti-TNF therapies (in particular antibodies to TNF, but not etanercept) may reset an imbalance between Th17 and Treg cells, and induce Treg cells capable of suppressing Th17 responses.
Treg cells at the inflamed site colocalize with DCs in the lymphoid aggregate areas of inflamed synovium, but it is thought that this colocalization cannot fully suppress DC activation and function. Interactions between Treg cells and DCs at sites of inflammation may lead to Treg cell proliferation. Murine studies have identified a feedback loop between Treg cells and DCs, regulated by Fms-like tyrosine kinase 3 ligand (Flt3L). Flt3L increased the number of DCs, which induced Treg cell division via MHC class II expression on DCs. This increase in DCs and Treg cells prevented the onset of autoimmune disease in mice. It has been reported that Flt3L levels are elevated in the synovial fluid (SF) of arthritis patients, and administration of Flt3L can alleviate arthritis in the methylated bovine serum albumin (mBSA) model. These findings indicate that Flt3L may act as one important regulator of the immune system via the expansion of DCs and Treg cells.
Monocytes are important players in the synovial microenvironment of autoimmune arthritis. Large numbers of activated monocytes are present in the inflamed joints and contribute to inflammation via secretion of a number of proinflammatory cytokines (e.g., IL-1β, IL-6, IL-7, and TNF-α). These cytokines can potentially affect the differentiation and function of Treg cells. For instance, IL-1β plays a role in the conversion of human Treg cells into IL-17–producing cells and IL-7, whereas TNF-α may abrogate Treg cell function. Also, IL-6, along with an unknown TLR-induced factor, renders effector T cells resistant to Treg-mediated suppression. In JIA the effector resistance demonstrated in synovial T-effector (Teff) cells could be partly replicated by pretreatment of Teff cells with IL-6/TNF. In RA, it has been reported that IL-6, TNF-α, and IL-1-β secreted by synovial monocytes induce expression of both proinflammatory (IL-17, IFN-γ, and TNF-α) and antiinflammatory (IL-10) cytokines by Treg cells. These Treg cells maintain their regulatory phenotype and have an enhanced suppressive ability; they are capable of suppressing IL-17 production. Thus it is possible that despite high levels of proinflammatory cytokines at sites of inflammation, Treg cells may still function effectively.
Fibroblast-like synoviocytes (FLS) are present within the synovial membrane. These cells display an altered phenotype in RA and produce a number of inflammatory mediators including matrix metalloproteases, chemokines, and cytokines. FLS play a major role in RA pathogenesis through the destruction of cartilage and bone and perpetuation of the inflammatory immune response. FLS express the T-cell growth factor IL-15 on their surface, which further stimulates effector T cells to produce proinflammatory cytokines. However, a recent study reported that FLS also induced the proliferation of Treg cells and enhanced their suppressive activity. IL-15 produced by FLS appears to have a dual effect on the balance between Treg cells and effector T cells. It has been reported that FLS from mice with zymosan-induced arthritis express the ligand for glucocorticoid-induced TNF receptor (GITR) and reduce the expression of GITR and FOXP3 in Treg cells via cell-to-cell contact. Furthermore, this interaction increased IL-6 production from FLS, which may cause Treg cells to convert to IL-17–producing cells or inhibit Treg cell suppression. Therefore, the interaction between FLS and Treg cells diminishes the suppressive activity of Treg cells and enhances the proinflammatory activity of FLS, thereby leading to exacerbation of arthritis. To date there are few data about FLS in JIA.
Osteoclasts, cartilage, and bone destruction are key features of arthritis. Cytokines such as TNF-α, receptor activator of nuclear factor kappa-B ligand (RANKL), and IL-17 induce an imbalance between bone formation (osteoblast dependent) and bone resorption (osteoclast dependent) resulting in enhanced bone loss. It has been reported that Treg cells can suppress osteoclast formation and may therefore regulate bone homeostasis. Treg cells have been shown to inhibit the development of collagen-induced arthritis (CIA) in mice and reduce the differentiation of osteoclasts, possibly due to induction of cytokines that inhibit osteoclastogenesis. Moreover, Treg cells have also been shown to suppress inflammation and bone destruction in TNF-mediated arthritis in transgenic mice. However, the bone-protective effects of Treg cells did not reflect improved inflammation but rather had a direct effect on the osteoclasts and osteoclast-mediated bone resorption. Furthermore, adaptive Treg cells may be superior to naturally occurring Treg cells in their ability to suppress osteoclastogenesis, possibly because adaptive Treg cells are more resistant to IL-6–mediated Th17 conversion. Thus, Treg cells both suppress inflammation and autoreactivity, and also directly suppress bone destruction.
Condrocytes. Most studies focus on synovial inflammation and hyperplasia with inflammatory pannus, but there are not many studies on the loss of human chondrocytes itself. It is known that the loss of cartilage resulting in cartilage degradation and erosion is an important pathogenetic mechanism in rheumatic joint diseases. Thus far, chondrocytes have been interpreted as passive participators in inflammatory joint diseases that become damaged during inflammation. However, it is also known that IL-1α and IL-1β are able to induce various cytokines in chondrocytes. Hence, the possible involvement of chondrocytes in the inflammatory process and in the progression of inflammatory joint diseases, which results in disruption of cartilage repair mechanisms and consequently cartilage degradation, has to be considered.
Linking Immune Phenotypes to Clinical Phenotypes
Systemic JIA (sJIA) is characterized by features such as fever, rash, and serositis. Due to the pronounced activation of a patient’s innate immune system and the absence of any consistent association with autoantibodies or HLA, it is increasingly believed that sJIA may be a polygenic autoinflammatory syndrome (see Chapter 16 ). Recent understanding of the roles for IL-1 and IL-6 in pathogenesis of sJIA has translated directly into new and effective treatment pathways for sJIA, by the use of IL-6 and IL-1 blockade. Tocilizumab, an anti-IL-6 receptor antibody, has been highly effective in the treatment of sJIA. Anti-IL-1 treatment, either by use of the IL-1R antagonist (anakinra), or anti-IL-1 antibodies such as canakinumab, can also be effective. Some data have suggested the delineation of two subpopulations of this form of disease—one with a pronounced, complete response to IL-1 blockade and another that is resistant to treatment or has an intermediate response. The observation that peripheral blood of sJIA patients has a higher frequency of Th17 cells than controls is interesting given the role of IL-6 in generation of the Th17 cell.
Although the category oligoarticular JIA is clinically heterogeneous, the early onset, ANA-positive form is a well-defined disease that occurs almost exclusively in children and has consistent HLA associations. Patients may have high concentrations of positive ANAs and a high risk of developing chronic iridocyclitis. Based on the current JIA classification criteria from the International League of Associations for Rheumatology (ILAR), oligoarthritis can be distinguished into two categories: persistent oligoarthritis, in which the disease affects four joints or fewer, and extended oligoarthritis, in which more than four joints are affected after the first 6 months of disease. The immunological phenotype has been shown to differ between these two types of oligoarticular JIA, in that the enrichment of Treg cells in the synovial compartment is more marked in persistent than extended oligoarthritis, whereas the synovial enrichment of Th17 cells is more marked in in extended oligoarthritis than persistent oligoarthritis. Immunological differences between these two are detectable, in the synovial cells, prior to clinical extension, which may in the future translate to clinical tests that could assist in the prediction of extension.
Rheumatoid factor (RF)-positive polyarthritis, a relatively rare subtype of JIA, is the only form of JIA with positive antibodies to cyclic citrullinated peptides (CCPs), and shares HLA associations with adult-onset RA (see Chapter 17 ). Enthesitis-related arthritis (ERA) JIA shares features with spondyloarthropathy; many patients are HLA-B27 positive, and the disease can progress to affect sacroiliac joints in about 30% to 40% of patients. Interestingly, ERAP-1 and IL23-R, genes associated with adult AS, are also associated with ERA (see Chapter 19 ).
RF-negative polyarthritis is a heterogeneous category of JIA. At least two subsets can be identified in this category: one that is similar to adult-onset RF-negative RA (characterized by a symmetric synovitis of large and small joints, onset at school age, and the absence of ANA expression), and another that resembles oligoarthritis. Similarities between this second subset and early-onset oligoarthritis has led some to suggest that pathological mechanisms may be similar in all children with early-onset, ANA-positive arthritis, irrespective of joint count. A major effort is underway to understand the molecular mechanisms a leading to resistance to therapy, for example, in severe JIA.
Translation Into Clinical Practice
Application of Modern Methods to Immune Modulation
The rapid evolution of multiplex, high-throughput technologies (HTT) (genomics, transcriptomics, proteomics, and metabolomics, among others) has provided the opportunity to probe large panels of candidate biomarkers. There are some cases of HTT research yielding a candidate, which can then be combined with traditional clinical laboratory markers to provide a composite score for use in the management of autoimmune disease. However, clinical application of various HTT is limited due to technology. At present, use of single nucleotide polymorphisms (SNPs) and other genetic markers as prognostic biomarkers are constrained by the multifactorial etiology of arthritis. Also, environmental triggers, stochastic events, and epigenetic marks are not captured by genetics. The intrinsic instability of candidate markers that exhibit high biological turnover rates, such as mRNA, and the operator- and procedure-dependent variability of plasma-derived candidates jeopardizes their translation to clinical settings. Proposed synovial candidates require invasive procedures and are therefore less practical, other than where joint aspiration is routine, as in oligoarticular JIA, which supports the case for reliable blood-based assays. In the future a combination of biomarkers, including protein, metabolome, and genetic tests may be used to reclassify diseases and provide prognostic algorithms, as is already the reality in oncology.
The recent advent of biologics, including TNF and other cytokine inhibitors, costimulation blockade and cell-depletion therapies has raised therapeutic expectations for treatment of childhood arthritis from the control of signs and symptoms to a goal of complete remission. However, these objectives are met in only one out of three patients. As of now, patients who will respond to the treatment are phenotypically indistinguishable from those who will not, leaving “trial and error” as the only feasible approach to determining the most effective treatment regimen. Thus, many patients remain exposed to the damage of continued active disease and to the costs and potential side effects of these drugs, with no substantial benefit. Across all autoimmune diseases, the identification of biomarkers that predict responsiveness to therapy is a major unmet medical need. All told, new developments in genetics, immunology, and imaging are instrumental to better define, classify, and treat children with autoimmune rheumatological diseases.
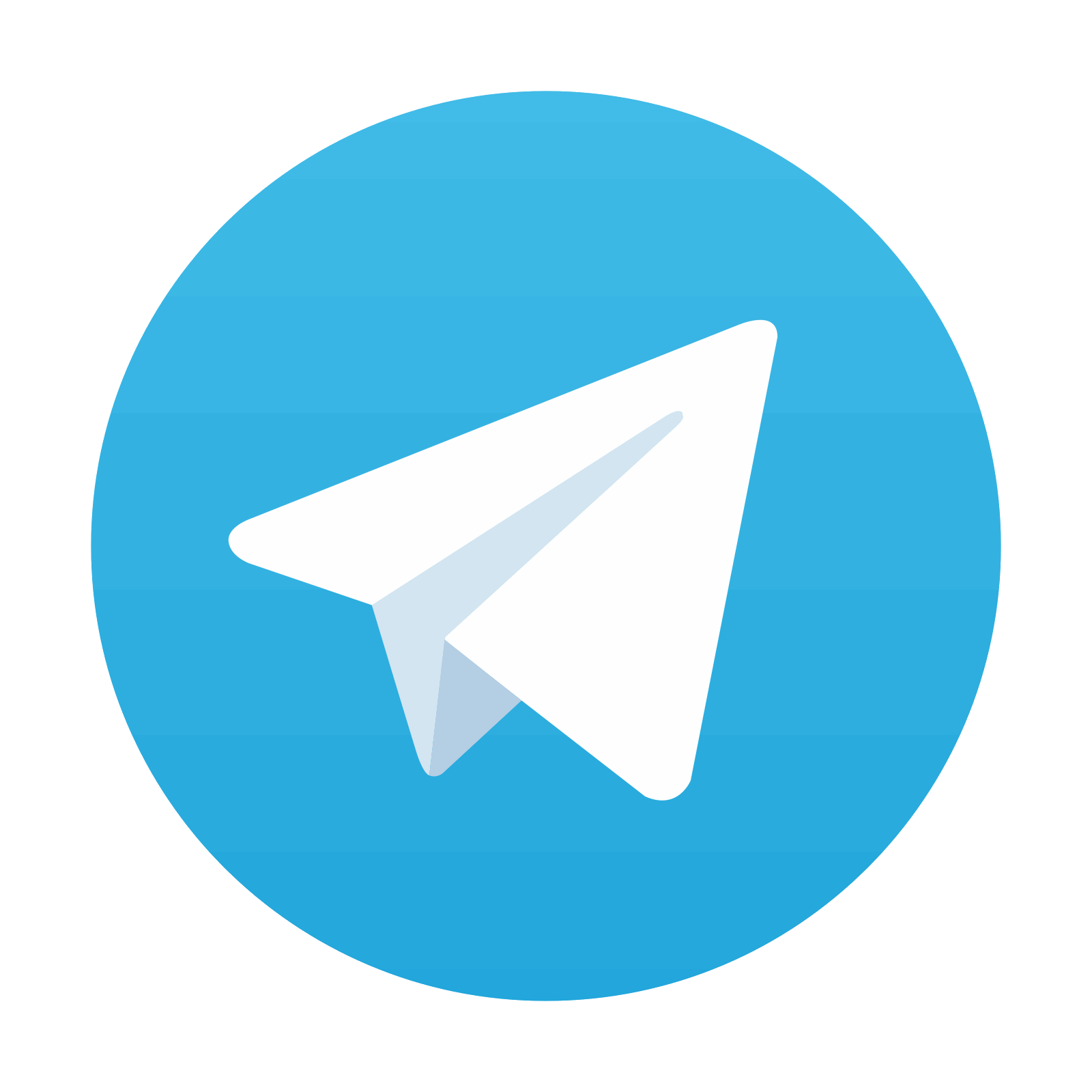
Stay updated, free articles. Join our Telegram channel

Full access? Get Clinical Tree
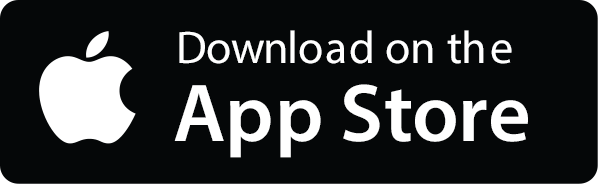
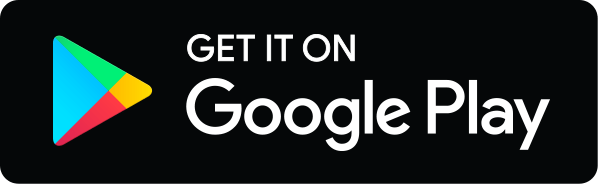