Participation in sports carries an inherent risk of head and neck injury. A relatively larger ratio of head to body places children at further risk for injury. This ratio decreases as children approach adolescence. Injuries to the head include scalp lacerations, skull fractures, brain injuries, and intracranial bleeding.
Of the 6000 neck injuries that occur annually among children a quarter of these are related to sports. High-risk sports are football, rugby, ice and field hockey, soccer, diving, gymnastics, cheerleading, and wrestling. Sports-related catastrophic neck injuries resulting in paralysis are rare with a prevalence of 2 per 100,000.1–7 Head and neck injury risk has long been a concern and cause for injury surveillance in contact sports such as football and hockey. As a result, changes in rules and techniques have helped to decrease injury rates. The National Collegiate Athletic Association (NCAA) injury surveillance system recently reported an injury rate of 2.34 and 0.61 per 1000 athlete-exposures (AE) for head and neck respectively in football.3 These rates were the highest of all sports. Other sports with significant risk were wrestling (1.27 AE head, 0.39 AE neck), lacrosse (1.08 AE head, 0.12 AE neck), and gymnastics (0.4 AE head, 0.28 AE neck). Hockey had an injury rate of 1.47 AE for the head and no significant neck injuries, owing to rule changes regarding checking from behind. While most head injuries were concussions, most neck injuries were strains.
Most sports-related skull fractures occur in the frontal and parietal bones. These fractures can be classified as either linear or depressed.6–9
Low-energy blunt trauma over a wide area of the skull can result in a linear fracture. Most of the fractures seen in children are a result of falls and bicycle accidents. These fractures involve the entire thickness of bone and can continue through the vasculature, resulting in the epidural hematoma. Contact of the skull with a projectile, such as a baseball, to the temple can result in a depressed fracture of the frontal or parietal bones. These fractures result from high-energy direct blow to a relatively small surface. The fracture may be comminuted and either open or closed. Since open fractures may need surgical evaluation and management, it is important to evaluate the athlete for associated laceration.
While loss of consciousness can occur, these athletes are often lucid. Concussion symptoms can present later. Other complications such as seizures can also occur. Epidural bleeding can be subtle and result quickly in worsening neurological function and even death. For this reason, any athlete who sustains this type of injury should be observed closely.
The imaging study of choice for severe head injury is a CT scan. Skull CT scan will confirm the fracture and also show evidence of bleeding or brain injury. The scan should be thin sliced bone windows. If bloody fluid is present in the nose or ear, a paper tissue maybe used to identify presence of cerebrospinal fluid. The “ring” sign is indicated when a halo of fluid is present on the tissue beyond the blood. A positive “ring” sign should raise suspicion for a basilar skull fracture.
Generally, outcome of skull fracture is good in the absence of neurological symptoms. In most cases without neurological signs, observation for 24 hours is all that is necessary. Patients with open fractures, evolving symptoms, and persistent symptoms should be referred to neurosurgery. Return to play should be delayed until the skull fracture has fully healed and patient is asymptomatic.7–9
Trauma of the head can lead to bleeding between the dura and the skull in the epidural space resulting in an epidural hematoma.7–9
As the bleeding expands, intracranial pressure is increased resulting in death in up to 20% of patients. The acceleration–deceleration mechanism is thought to cause shearing which results in tearing of blood vessels in the epidural space. Fractures from a blunt trauma can directly tear superficial arteries.
Because it is often bleeding from arteries, hemorrhage can occur quickly. Athletes with epidural bleeding can potentially progress to death within 6 to 8 hours.8 They can be quite lucid and symptom-free during this period. A subtle progressive headache may be the initial sign. As the brain stem becomes compressed, the athlete will demonstrate decreased pupil response, decreased consciousness, and abnormal posturing.
CT scan is the study of choice although the hematoma may be seen on MRI as well (Figure 34-1). There is often association of epidural hematoma and subdural hematoma and both may be seen on scans.
Blood collecting between the dura and the arachnoid space is known as subdural hematoma, and can be acute or chronic. Acute subdural hemorrhage accounts for most deaths caused by sport-related head trauma.
Following head trauma the bridging veins in the subdural space can tear and result in bleeding. Compared to the epidural bleeding which results from arterial injury and forms quickly, subdural bleeding forms more slowly. Thus, the course and progression are slower.
Like the epidural hematoma there is a high mortality rate associated with the subdural hematoma. Mortality related to subdural hematoma can approach 60% to 80%.7–9 Symptoms may have a more gradual onset over days rather than hours as is seen with epidural hematomas. Often neurological symptoms such as numbness, headache, disorientation, amnesia, inability to concentrate, ataxia, lethargy, nausea, vomiting, and slurred speech are present. Occasionally, seizures may occur. Sometimes only subtle personality changes are present. Many of these symptoms are similar to those seen with concussion and athlete with persistent post-concussion symptoms should be evaluated for subdural bleed.
Subdural hematoma should be visible on CT scan. MRI will show changes as well, though the imaging study of choice is the CT scan (Figure 34-2). Bleeding disorders should be considered in the differential diagnosis. Athletes may be at greater risk if taking aspirin or NSAIDs which can interfere with blood clotting.
In subarachnoid hemorrhage the blood collects in the subarachnoid space, between the arachnoid and pia mater as a result of blood vessel or brain parenchyma injury. This is a very rare occurrence in sport-related head trauma.
Trauma to the blood vessels in the pia mater or in the brain can lead to leakage of blood in the subarachnoid space.
Headache is usually associated with subarachnoid hematoma. The same neurological symptoms of nausea, vomiting, confusion, loss of consciousness, or even seizure are associated with this bleeding. Intraocular hemorrhage may occur. Progression can be rapid with a 50% overall survival, with 10% to 15% mortality before arriving to the hospital.7–9
CT scan or MRI may show subarachnoid bleeding. Occasionally, lumbar puncture is required to identify the bleeding. Vascular anatomy should be evaluated by either CT scan or MR angiogram. A cerebral aneurysm may be present as an underlying cause.
Head trauma can cause bleeding within the brain tissue itself. Coagulation abnormalities should be considered as well as possible aneurysms or arteriovenous malformation. Rapidly progressive neurological symptoms such as headache, ataxia, slurred speech, weakness, inability to concentrate, and confusion will be present. CT scan can often identify the bleeding within brain tissue. Treatment is supportive, with referral to neurosurgery.
The scalp can be lacerated by contact typically encountered with sports, even in noncontact sports. If there is a significant blunt trauma to the head, more serious underlying injuries such as a skull fracture or serious neurological compromise because of bleeding should be ruled out. The laceration may be associated with an underlying open skull fracture.
Blood flow to the scalp is very good and even small lacerations can bleed extensively. The presence of scalp hair can interfere with visualization. Anesthesia with lidocaine with epinephrine can relieve pain and decrease bleeding to allow adequate evaluation. In children, this may be difficult. Most scalp lacerations are superficial and are closed easily. Rarely, deeper sutures are required to allow for closure. Staples are a widely used option for closure of the scalp laceration. If the edges are smooth and regular, adhesive could be used, otherwise, nonabsorbable suture works well. Since these lacerations are usually dirty, irrigation with 200 cm3 normal saline will adequately cleanse the site. Healing is usually rapid and sutures or staples may be removed as early as 1 week.
It is estimated that there are 1.4 million high-school and college-age players.1–3 Cervical spine injuries affect 15% of these players.1–3 Between 1977 and 1989, 128 players suffered a permanent spinal cord injury.1–3,7 This translates to about 10 spinal cord injuries per year. Half of college football players with neck injury showed x-ray changes. Worldwide, the sport of rugby has the highest risk of neck injury. A retrospective descriptive case series study of cervical spinal injury (CSI) in school-aged children injured in community-based rugby football reported 125 children with CSI, most (97%) were boys.
Serious cervical spine fractures are most likely to occur with the head flexed to 30 degrees while axially loaded from the top of the head.7 This position tends to place the cervical spine in a straight column (Figure 34-3). An axial load to the column will result in failure or the burst fracture at mid-column (C3-C5). This results in not only an unstable spinal column, but fracture fragments can transect the spinal cord resulting in permanent quadriplegia. Rule changes making the use of the head as an initial point of contact in football illegal, has decreased spinal injuries. Instructing athletes in football and hockey to keep their head up during contact has also decreased catastrophic neck injuries.
Figure 34-3

Mechanism of cervical spine failure and fracture. Cervical spine normally maintains a slight lordosis. In this alignment and in neutral alignment (A) a compressive axial force is more effectively dissipated by the muscles and ligaments of cervical spine and neck. When the cervical spine sustains an axial load in a flexed position (B), it tends to buckle and deform resulting in fracture and dislocation (C, D).
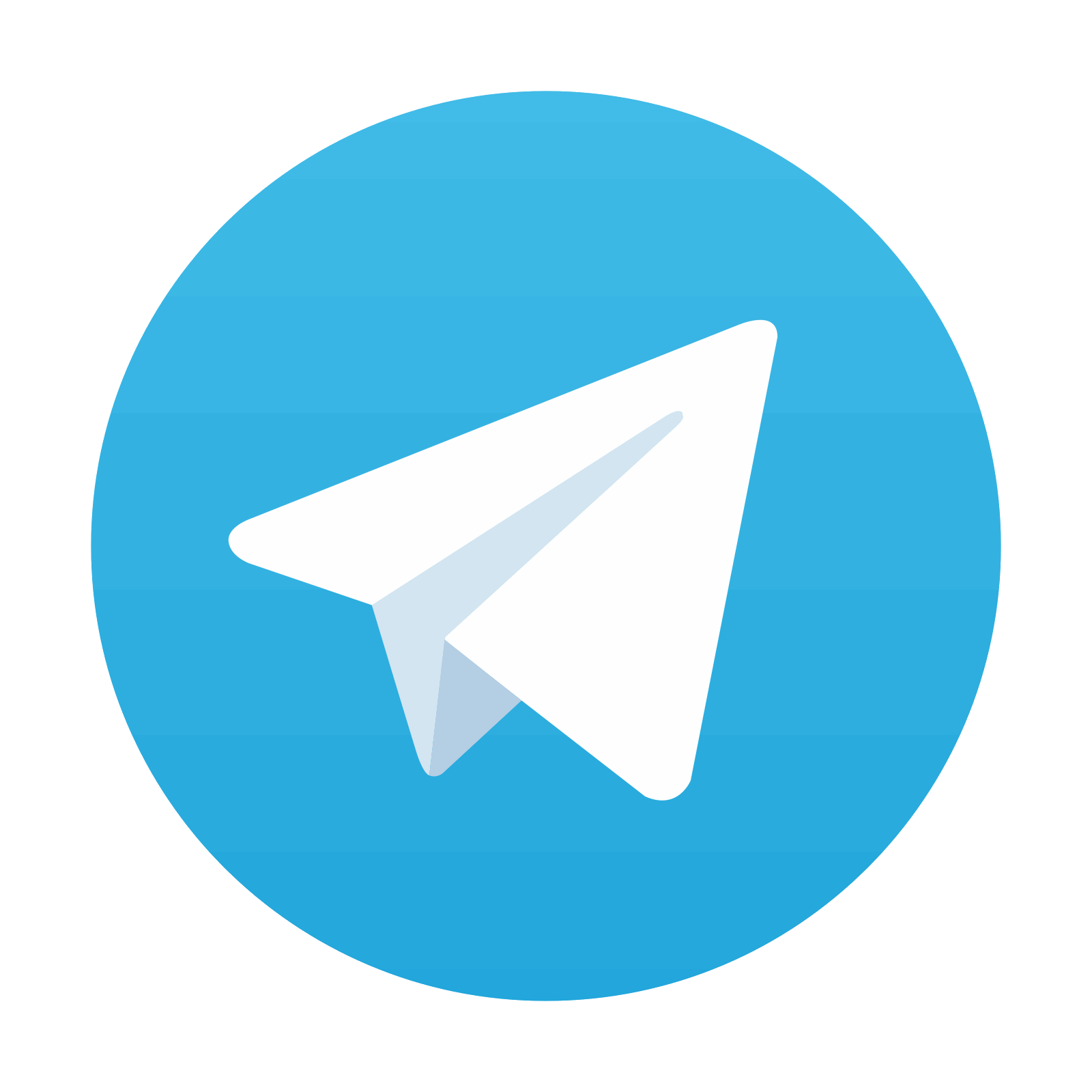
Stay updated, free articles. Join our Telegram channel

Full access? Get Clinical Tree
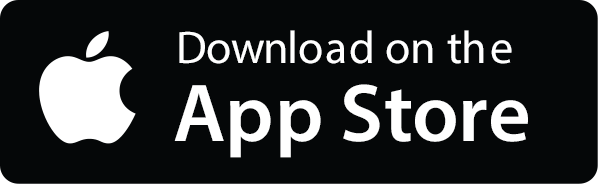
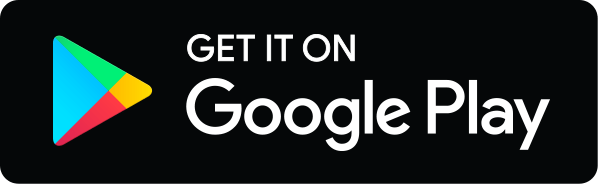
