Small-molecule kinase inhibitors are increasingly taking center stage in the quest for new drugs for the treatment of rheumatoid arthritis (RA). By targeting kinases, small-molecule inhibitors can exert potent anti-inflammatory and immunomodulatory effects; the success of small-molecule kinase inhibitors in the treatment of cancer has spurred efforts to identify kinases that could be targeted for the treatment of chronic inflammatory disorders, such as RA. Although many kinase inhibitors have proved efficacious in the treatment of inflammatory arthritis in animals few have been tested in RA clinical trials. This article discusses the challenges and progress in the pursuit of small-molecule kinase inhibitors for RA, including lessons learned from the failure of erstwhile frontrunner inhibitors and the promise of inhibitors making their debut on the RA stage.
The advent of biologic therapeutics, most notably anti–tumor necrosis factor (TNF) agents, has dramatically improved the treatment of rheumatoid arthritis (RA). Nevertheless, the available biologics rarely result in disease remission and provide clinical benefit only in subsets of RA patients. In addition, biologics can be administered only by injection and are expensive. Alternative therapies for RA are needed, and small-molecule kinase inhibitors may fit the bill. Small molecules have several features that give them the edge over other therapeutics: they are orally bioavailable, cell permeable, and inexpensive to manufacture. Insight into intracellular signaling pathways involved in inflammation and immunity has allowed the rational design of small molecules that can counteract aberrant immune responses. Small molecules can exert potent anti-inflammatory effects by inhibiting kinases, many of which lie at the nexus of multiple proinflammatory pathways. The therapeutic potential of kinase inhibitors is showcased by their success in the treatment of cancer.
Adaptive and innate immune responses are involved in the pathogenesis of RA, a systemic autoimmune disease characterized by destruction of the synovial joints. Initiation of the disease involves systemic dysregulation of T- and B-cell responses, which leads to a breach in self-tolerance and eventually to the mounting of an immune response against the synovial joints. During the chronic inflammatory stage of the disease, mast cells, macrophages, neutrophils, T cells, and B cells all infiltrate the synovium, where they release proinflammatory cytokines and matrix metalloproteinases (MMPs) that erode the synovial cartilage. Inflammation in the joints also triggers the development of apoptosis-resistant, hyperproliferative, fibroblast-like synoviocytes (FLS), which produce further proinflammatory cytokines. The synovial hyperplasia, in turn, leads to the formation of a destructive pannus that invades surrounding cartilage and bone. Finally, inflammation suppresses the formation of bone-forming osteoblasts and augments the formation of bone-resorbing osteoclasts, leading to the erosion of bone. Several kinases have been shown to play important roles in one or more of these pathogenic processes.This article discusses the therapeutic potential of small molecules targeting specific protein kinases in the treatment of RA and provides an overview of the progress to date. Lipid kinases—in particular, the phosphoinositide-3 kinases (PI3Ks)—are also emerging as attractive drug targets in the treatment of inflammation. The therapeutic potential of blocking PI3Ks in RA has been recently reviewed and is not discussed further.
Mitogen-activated protein kinases: advances and setbacks
Mitogen-activated protein kinase (MAPK) signaling comprises 3 interrelated pathways, each mediated by a distinct MAPK: p38, extracellular signal-regulated kinase (ERK), or c-Jun N-terminal kinase (JNK). These pathways involve the sequential activation of multiple kinases, such that the MAPKs are activated by MAPK kinases (MKKs), which are themselves activated by MAPKK kinases (MKKKs). Thus, the p38 kinases (α, β, γ, and δ) are activated by MKK3 and MKK6, the ERKs (1 and 2) by MAPK-ERK kinase (MEK) 1 and MEK2, and the JNKs (1, 2, and 3) by MKK4 and MKK7. JNK, ERK, and p38 are the terminal kinases of these pathways and serve to regulate an array of cellular responses through the phosphorylation of serine/threonine residues in discrete sets of transcription factors. All 3 of these MAPKs are activated in RA synovium and have been proposed as therapeutic targets in the treatment of RA.
P38
Enthusiasm for inhibitors of p38—until recently heralded as one of the most promising classes of oral therapeutics for RA—has finally subsided. Many p38 inhibitors have been developed and tested in preclinical and clinical studies. Although the preclinical data were encouraging, with p38 inhibition shown to suppress inflammation and joint destruction in many different models of RA, these initial successes did not extend to the treatment of RA. The first generation of small-molecule p38 inhibitors, which targeted all 4 isoforms of p38, failed in clinical trials owing to liver, brain, and skin toxicities. Nevertheless, the discovery that p38α is the important isoform in RA, acting to drive the expression of proinflammatory cytokines and the formation of osteoclasts, engendered hope that selective inhibition of p38α would avoid the adverse effects of the pan-38 inhibitors. Unfortunately, p38α-specific inhibitors did not perform much better ( Table 1 ). For instance, clinical development of SCIO-323 and AMG-548 was terminated because of skin toxicity and liver toxicity, respectively, and the p38α inhibitors that did advance to phase II clinical trials proved ineffective.
Kinase Target | Inhibitor (Company) | Outcome in RA Clinical Trials |
---|---|---|
p38α | SCIO-323 (Scios) AMG-548 (Amgen) Pamapimod (Roche) VX-702 (Vertex) |
|
JAKs | INCB18424 (Incyte) CP690550 (Pfizer) |
|
Syk | R788 (Rigel) |
|
MEK 1/2 | ARRY-162 (Array BioPharma) |
|
c-Kit/PDGFR | AB1010 (AB Science) Imatinib mesylate (Novartis) |
|
a Incyte press release. Incyte’s JAK inhibitor demonstrates marked clinical benefits in phase IIa rheumatoid arthritis study. Available at: http://www.incyte.com/Eular%20PR%206%2012%2008%13-%20Final.pdf . Accessed January 25, 2010.
b Array BioPharma. Array Biopharma announces top-line results from rheumatoid arthritis phase 2 trial. Available at: http://www.drugs.com/clinical_trials/array-biopharma-announces-top-line-results-rheumatoid-arthritis-phase-10-trial-7991.html . Accessed January 25, 2010.
c Novartis Pharmaceuticals. A study of imatinib 400 mg once daily in combination with methotrexate in the treatment of rheumatoid arthritis. Available at: http://www.clinicaltrials.gov/ct2/show/NCT00154336?term=imatinib+and+rheumatoid&rank= . Accessed January 25, 2010.
The toxicity and the inefficacy of p38 inhibitors are most likely target based, rendering the systemic targeting of p38 unviable. Multiple structurally unrelated p38 inhibitors have been shown to be toxic to the liver and skin and to induce only transient reductions in markers of inflammation. The pivotal position of p38α in the regulation of inflammation is thought to underlie these phenomena. Although its proinflammatory role has long been recognized, p38α has more recently been found to play an anti-inflammatory role also. Not only does it drive the expression of important anti-inflammatory genes but also it mediates intracellular feedback loops that constrain the activity of other proinflammatory pathways. For instance, p38α activates mitogen- and stress-activated protein kinase (MSK) 1 and MSK2, which contribute to the resolution of inflammation through the transcriptional activation of anti-inflammatory genes, such as interleukin (IL)-10, IL-1 receptor antagonist, and protein phosphatase dual specificity. p38α also reigns in inflammation by phosphorylating TGF-beta-activated kinase (TAK) -associated kinase 1 (TAB1), thereby inhibiting TAK1, which regulates the proinflammatory JNK and inhibitor of κB (IκB) kinase (IKK) pathways, as well as p38α itself. Thus, blockade of p38α would allow inflammation to proceed unchecked. Genetic evidence supports the idea that p38α inhibition underlies the toxicity and inefficacy of p38 inhibitors: myeloid cell-specific ablation of p38α in mice results in increased ERK and JNK activity and in vascular permeability and edema ; double deficiency in MSK1 and MSK2 leads to prolonged inflammation in a model of toxic contact eczema ; and hepatocyte-specific ablation of p38α in mice results in excessive activation of the proapoptotic JNK in the liver after lipopolysaccharide (LPS) challenge.
Although the death knell may have sounded for inhibitors of p38, components downstream of p38α may yet constitute viable therapeutic targets. MAPK-activated protein kinase 2 (MK2), a kinase downstream of p38α that post-transcriptionally promotes the expression of proinflammatory genes, has been proposed as one such candidate. Targeting of MK2 should spare p38α-mediated anti-inflammatory mechanisms, including the p38α-TAB1 feedback loop and expression of anti-inflammatory genes. Support for such an approach comes from the finding that MK2-deficient mice are protected against collagen-induced arthritis (CIA). One small-molecule MK2 inhibitor has already been shown to reduce LPS-induced TNF production in rats, and many more are being synthesized.
MEKs and ERKs
The critical role of MEK-ERK signaling in cell proliferation has led to MEK1 and 2 and ERK1 and 2 being investigated as candidate targets in clinical trials in cancer. MEK-ERK signaling is upregulated in synovial tissues in RA and in CIA and promotes proliferation of RA FLS in vitro. MEK-ERK signaling may thus contribute to the pathogenesis of RA by driving formation of the tumor-like pannus that is characteristic of RA. But the MEK-ERK cascade is not solely a proliferative one—it is also proinflammatory, inducing the production of IL-1β, IL-6, TNF, and MMPs, and itself is activated by proinflammatory cytokines. In addition to promoting inflammation and tissue destruction in the synovial joints, ERK signaling is important in lymphocyte activation and differentiation. ERK mediates B-cell receptor (BCR) and CD40 receptor signaling in B cells and T-cell receptor signaling in T cells. Recent data suggest that ERK dysregulation in CD4 + and CD8 + T cells may even contribute to the breakdown of T-cell tolerance in RA by lowering the threshold for T-cell activation.
Several small-molecule inhibitors of MEK 1 and 2 have shown efficacy in animal models of RA ( Table 2 ). Oral administration of PD184352 to mice with CIA suppressed synovitis, pannus formation, and cartilage and bone erosion. These effects correlated closely with the inhibition of ERK phosphorylation in mouse joints. PD184352 also prevented proteoglycan loss in articular cartilage in a rabbit model of IL-1β-induced arthritis. Prophylactic, intraperitoneal administration of subtherapeutic doses of U0126 to SKG mice, which spontaneously develop autoimmune arthritis owing to a mutation in zap70 , delayed disease onset and reduced disease severity, supporting the concept that ERK dysregulation may contribute to the development of RA. A third MEK1 and 2 inhibitor, ARRY-162, inhibited inflammation and bone resorption in mice with CIA and in rats with adjuvant-induced arthritis (AIA) and exhibited additive efficacy when combined with standard-of-care agents such as anti-TNFs and methotrexate. These promising findings saw ARRY-162 enter clinical development; however, despite being well tolerated, ARRY-162 did not fare any better than placebo in a recent phase II, 12-week trial in patients with active RA on a background of methotrexate treatment (see Table 1 ).
Kinase Targeted | Small-Molecule Inhibitor (Company) | RA Model |
---|---|---|
MEK 1/2 ERK 1/2 | PD184352 (Pfizer) U0126 (DuPont Pharmaceuticals) ARRY-162 a (Array BioPharma) FR180204 (Astellas) | Mouse CIA; rabbit cytokine-induced cartilage degradation Mouse SKG Rat CIA and AIA Mouse CIA |
JNK 1/2/3 | SP600125 (Celgene) AS601245 (EMD Serono) | Rat AIA Mouse CIA |
IKK2 | BMS-345541 (Bristol-Myers Squibb) BMS-066 (Bristol-Myers Squibb) TPCA-1 (GlaxoSmithKline) PHA-408 (Pfizer) ML120B (Millenium Pharmaceuticals) IMD-0560 (IMDD) SPC839 (Celgene) | Mouse CIA Mouse CIA and rat AIA Mouse CIA Rat SCWA Rat AIA Mouse CIA Rat AIA |
c-Fms | GW2580 (GlaxoSmithKline) Ki20227 (Kirin) Cyanopyrrole 8 (Johnson & Johnson) | Rat AIA ; mouse CIA, CAIA, K/BxN Mouse CIA Mouse CIA |
BTK | Compound 4 (Celera Genomics) Cgi1746 (CGI Pharmaceuticals) | Mouse LPS-induced arthritis Mouse CIA |
PI3Kγ | AS-604850, AS-605240 (EMD Serono) | Mouse CAIA |
a In clinical trials in RA patients (see Table 1 ).
In addition to inhibitors of MEK1 and 2, an inhibitor of the downstream ERKs has been assessed in a mouse model of RA. Intraperitoneal administration of the ERK 1 and 2 inhibitor FR180204 to mice before the induction of CIA reduced the clinical signs of arthritis, the production of anti-collagen type II (CII) antibodies, and CII-specific proliferation of T cells. Conversely, recent studies suggest that targeting components upstream of MEK may also provide efficacy in RA. Tumor progression locus 2 (TPL2) is the MKKK that activates MEK1 and 2 and hence the ERKs. Studies using tpl2 −/− mice have shown that TPL2 is required for LPS-induced production of circulating TNF in vivo and for LPS-induced production of TNF by macrophages in vitro. Furthermore, TPL2 deficiency has been shown to protect mice from TNF-induced inflammatory bowel disease and arthritis (unpublished data with G. Kollias cited in Das and colleagues ). Several small-molecule inhibitors of TPL have been assessed for their ability to suppress TPL2-MEK-ERK–induced inflammation. Compound 1 suppressed LPS- and IL-1β–induced production of TNF by human monocytes as well as IL-1β–induced production of IL-6, IL-8, prostaglandin E2, and MMPs by RA FLS. Compound 44 inhibited the production of TNF in an LPS-induced model of inflammation in rats. Results from the testing of TPL2 inhibitors in animal models of RA have not been described to date.
Thus, small-molecule inhibitors exist for the targeting of the TPL2-MEK-ERK pathway at 3 different levels. The inefficacy of the MEK1 and 2 inhibitor ARRY-162 in a phase II RA trial, however, together with concerns that MEK/ERK inhibition could result in the development of lupus-like disease, raise doubts over the potential of MEK/ERK inhibitors for the treatment of RA. Safety might also be an issue with TPL2 inhibitors, but these could potentially provide greater therapeutic efficacy than MEK/ERK inhibitors. Although the signaling defect in TPL2-deficient macrophages and B cells seems restricted to activation of the MEK-ERK pathway, TPL2 regulates the activation of JNK and nuclear factor κB (NF-κB), in addition to ERK, in mouse embryonic fibroblasts. Because synovial-fibroblast production of proinflammatory and degradative mediators is important in the pathogenesis of RA, inhibition of TPL2 might provide added benefit by suppressing ERK-driven activation of lymphocytes and ERK-, JNK-, and NF-κB–driven activation of synovial fibroblasts.
JNKs
Activated by stress signals and cytokines, JNKs play important roles in apoptosis, inflammation, and matrix degradation. JNKs exist as 3 isoforms: JNK1, JNK2, and JNK3. JNK1 and JNK2 are ubiquitously expressed, and phosphorylation of these isoforms is detected in RA synovium but not in osteoarthritic synovium ; JNK3 expression is largely restricted to the brain, heart, and testes, and, therefore, not thought to be involved in RA. As discussed later, some of the efficacy of spleen tyrosine kinase (Syk) inhibitors in RA could potentially be attributed to the inhibition of JNKs, because the tyrosine kinase Syk lies upstream of JNK in the MAPK signaling cascade. Syk-activated JNKs drive the expression of IL-6 and MMP-3 in RA FLS. Induction of MMP expression is defective in JNK1- or JNK2-deficient murine FLS, and pharmacologic inhibition of JNK blocks induction of MMP expression in RA FLS. In addition to promoting synoviocyte production of proinflammatory mediators, JNK1 regulates the differentiation of T cells into Th1 cells.
The JNK-driven expression of MMPs seems critical in the destruction of joints in inflammatory arthritis. Subcutaneous administration of SP600125, a small-molecule inhibitor that targets all 3 JNK isoforms, suppressed cartilage and bone erosion in rat AIA, effects associated with inhibition of JNK activity and MMP expression in the joints. Oral administration of another pan-JNK inhibitor, AS601245, attenuated CIA in mice, reducing synovial inflammation and cartilage degradation. JNK1 deficiency, however, does not confer resistance to destructive arthritis in JNK1-deficient, TNF-transgenic mice nor does it reduce the activity of JNK-mediated signaling. In addition, JNK2 deficiency confers only modest protection against the development of anti–collagen antibody-induced arthritis (CAIA). Together, these findings suggest that inhibition of both JNK1 and JNK2 is required for the effective attenuation of inflammatory arthritis.
Although developed as a JNK inhibitor, SP600125 has been shown to inhibit 13 other protein kinases with similar or greater potency and to have an unfavorable pharmacokinetic profile. Likewise, AS601245 exhibits only moderate selectivity for JNK. More specific inhibition of the JNK signaling cascade can be achieved by targeting the physical interaction between JNK and other components of the cascade. JNK-interacting protein 1 (JIP1) is a scaffolding protein that promotes JNK activity by facilitating the interaction between JNK and upstream kinases. Overexpression of JIP1, however, suppresses JNK activity (presumably owing to the sequestration of JNK-interacting components), and a peptide corresponding to the minimal region of JIP1 (pepJIP1) has been developed as an inhibitor of JNK. Although peptide therapeutics are associated with certain disadvantages, such as their rapid degradation in vivo and the need for administration via injection, a small-molecule mimic of pepJIP1, BI-78D3, was recently developed and shown to exert anti-inflammatory effects in vivo, restoring insulin sensitivity in a mouse model of type 2 diabetes. In addition, a small-molecule inhibitor that selectively blocks the DNA-binding activity of AP-1, an important JNK-activated transcription-factor complex, was recently shown to be efficacious in a mouse model of arthritis. Oral administration of the AP-1 inhibitor T-5224 prevented and treated CIA in mice, abrogating joint destruction and suppressing MMP and IL-1β expression.
Although toxicity in animal models treated with inhibitors of the JNK pathway has not been reported, long-term suppression of JNK could potentially have adverse effects due to JNK’s role in regulating apoptosis. JNK1-deficient mice spontaneously develop intestinal tumors and are more susceptible to the development of TPA-induced skin tumors. Thus, increased tumorigenicity may limit the value of JNK inhibitors for the treatment of chronic inflammatory disorders, such as RA.
JNKs
Activated by stress signals and cytokines, JNKs play important roles in apoptosis, inflammation, and matrix degradation. JNKs exist as 3 isoforms: JNK1, JNK2, and JNK3. JNK1 and JNK2 are ubiquitously expressed, and phosphorylation of these isoforms is detected in RA synovium but not in osteoarthritic synovium ; JNK3 expression is largely restricted to the brain, heart, and testes, and, therefore, not thought to be involved in RA. As discussed later, some of the efficacy of spleen tyrosine kinase (Syk) inhibitors in RA could potentially be attributed to the inhibition of JNKs, because the tyrosine kinase Syk lies upstream of JNK in the MAPK signaling cascade. Syk-activated JNKs drive the expression of IL-6 and MMP-3 in RA FLS. Induction of MMP expression is defective in JNK1- or JNK2-deficient murine FLS, and pharmacologic inhibition of JNK blocks induction of MMP expression in RA FLS. In addition to promoting synoviocyte production of proinflammatory mediators, JNK1 regulates the differentiation of T cells into Th1 cells.
The JNK-driven expression of MMPs seems critical in the destruction of joints in inflammatory arthritis. Subcutaneous administration of SP600125, a small-molecule inhibitor that targets all 3 JNK isoforms, suppressed cartilage and bone erosion in rat AIA, effects associated with inhibition of JNK activity and MMP expression in the joints. Oral administration of another pan-JNK inhibitor, AS601245, attenuated CIA in mice, reducing synovial inflammation and cartilage degradation. JNK1 deficiency, however, does not confer resistance to destructive arthritis in JNK1-deficient, TNF-transgenic mice nor does it reduce the activity of JNK-mediated signaling. In addition, JNK2 deficiency confers only modest protection against the development of anti–collagen antibody-induced arthritis (CAIA). Together, these findings suggest that inhibition of both JNK1 and JNK2 is required for the effective attenuation of inflammatory arthritis.
Although developed as a JNK inhibitor, SP600125 has been shown to inhibit 13 other protein kinases with similar or greater potency and to have an unfavorable pharmacokinetic profile. Likewise, AS601245 exhibits only moderate selectivity for JNK. More specific inhibition of the JNK signaling cascade can be achieved by targeting the physical interaction between JNK and other components of the cascade. JNK-interacting protein 1 (JIP1) is a scaffolding protein that promotes JNK activity by facilitating the interaction between JNK and upstream kinases. Overexpression of JIP1, however, suppresses JNK activity (presumably owing to the sequestration of JNK-interacting components), and a peptide corresponding to the minimal region of JIP1 (pepJIP1) has been developed as an inhibitor of JNK. Although peptide therapeutics are associated with certain disadvantages, such as their rapid degradation in vivo and the need for administration via injection, a small-molecule mimic of pepJIP1, BI-78D3, was recently developed and shown to exert anti-inflammatory effects in vivo, restoring insulin sensitivity in a mouse model of type 2 diabetes. In addition, a small-molecule inhibitor that selectively blocks the DNA-binding activity of AP-1, an important JNK-activated transcription-factor complex, was recently shown to be efficacious in a mouse model of arthritis. Oral administration of the AP-1 inhibitor T-5224 prevented and treated CIA in mice, abrogating joint destruction and suppressing MMP and IL-1β expression.
Although toxicity in animal models treated with inhibitors of the JNK pathway has not been reported, long-term suppression of JNK could potentially have adverse effects due to JNK’s role in regulating apoptosis. JNK1-deficient mice spontaneously develop intestinal tumors and are more susceptible to the development of TPA-induced skin tumors. Thus, increased tumorigenicity may limit the value of JNK inhibitors for the treatment of chronic inflammatory disorders, such as RA.
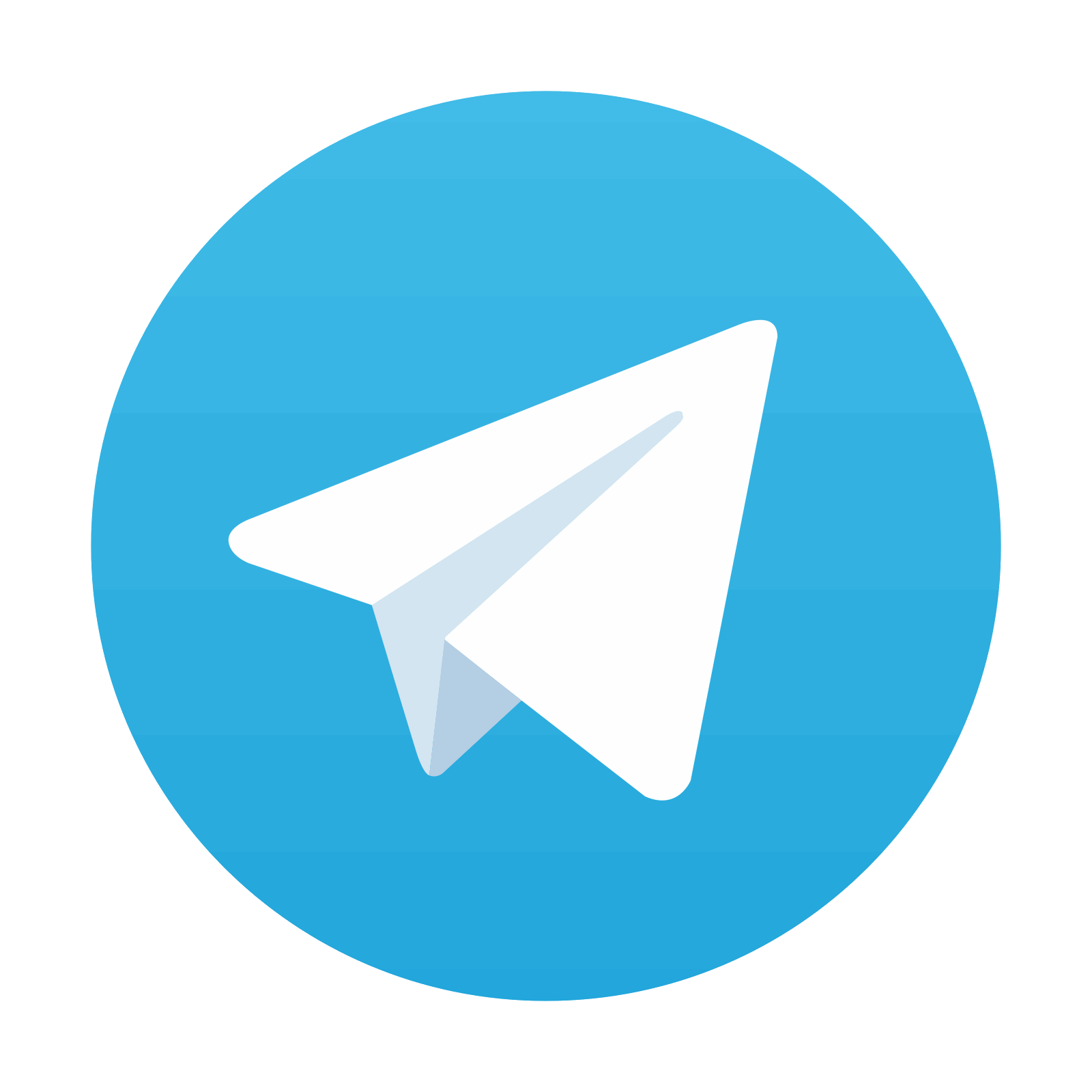
Stay updated, free articles. Join our Telegram channel

Full access? Get Clinical Tree
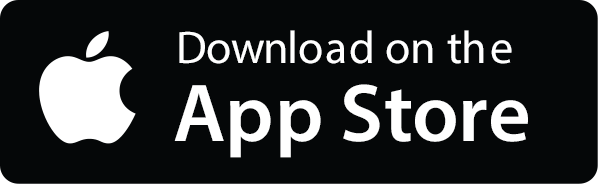
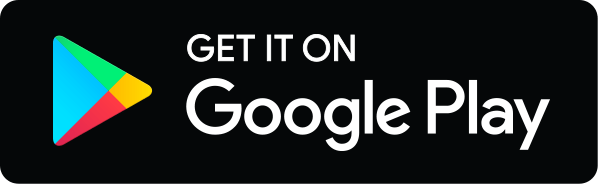